Evolution
Encyclopedia Vol. 1
CHAPTER EIGHT THE EARTH

Although many of its ecosystems were damaged by the
worldwide Genesis Flood, yet our planet remains wonderfully designed for
living. Later, in chapter 19, we will learn more about the effects of
the deluge. But now, for a few moments, let us consider some of the many
factors that make our world so livable. Because entire volumes could be
written on this topic, we will briefly focus our attention on three
topics: atmosphere, water, and soil.
CHAPTER 8 —
DNA AND PROTEIN EVOLUTION
BASIC ARRANGEMENT OF THIS
CHAPTER
Introduction
DNA and protein design voids the possibility of
evolution
1 — DNA code and structure
2 — Mathematics of DNA
3 — Amino acids and protein
4 — Experimental amino acid synthesis
5 — More problems with protein
6 — Originating the five basic materials
7 — Proteins and mathematics
Appendices
1 — Gregor Mendel's discovery
2 — Story of DNA
3 — Origin of DNA
4 — More mathematical impossibilities
5 — DNA, protein and the cell
6 — Amino acid functions
7 — Coding in the information
Related studies:
Chapter 7, Primitive Environment
Chapter 9, Cellular
1 - THE ATMOSPHERE
Ours has been called the "water planet;" it
is also the "air planet." These are two special qualities
about our world that are not to be found on any of the other planets in
our solar system.
The air surrounding our world is called the
atmosphere. Air has no color, smell, or taste, yet without it there
could be no living plants or animals on the earth. People are known to
have survived more than a month without food, and more than a week
without water. But without air they die within a few minutes.
Without air, there would be no weather. We could have
no wind, and no storms which bring us
much-needed water. Without wind there would be no movement of the trees
and plants and our world would be very still. It would also be
silent, for without air we could hear almost nothing. Most sound
travels through the air (although some travels through rock, metal, and
water.) Sound cannot travel in a vacuum.
Without air, birds could not fly. Air provides
resistance to motion, and it is this resistance which enables birds and
planes to fly through the air. Without air, there would be no clouds.
The sky would maintain a dreary blankness day after day. The sky
would not be blue; instead it would be black.
Air is composed of several invisible gases. About 98
percent of those gases are nitrogen and oxygen. Two-tenths of all the
air is composed of oxygen (21 percent). Without oxygen we could not
survive, for we need it continually in our blood and tissues. Plants
would quickly die without it also. They need it just as they need
carbon dioxide.
But eight tenths of the air is seemingly useless to
us; it is nitrogen (78 percent). Surely, it must have a purpose also;
everything else does. Actually, it is invaluable. Oxygen is combustible;
that is, it can be set on fire and burn. If there were no nitrogen in
the atmosphere, the world would have burned up as soon as the first
fire had been ignited by lightning, or the first two flinty rocks
striking one another had sparked. Even iron would have burned. We have
cause to be very thankful for the nitrogen in the air around us.
The remaining 1 percent of air consists almost
entirely of the gas argon. But there are also small amounts of neon,
helium, krypton, xenon, hydrogen, ozone, carbon dioxide, nitrous oxide,
and methane gases.
All those various gases are invisible. What if they
were even slightly opaque? Our world would be totally dark. The
gloom of eternal night would be upon us, even though the sun shined
brightly overhead. Ocean water looks fairly clear, but 200 feet [61 m]
down, the sunlight is nearly gone, and 300 feet [91 m] down darkness
prevails. The atmosphere over our heads is hundreds of miles deep and
covers all the earth. If the gases in it were not transparent, we would
all live in perpetual darkness. The world would be ice cold. The
warming rays of the sun would be blocked out before reaching us. The
tiny photosynthesis factories contained within each plant leaf could not
operate. No food would be produced, and all the plants and
animals would die.
There is also some dust in the air. This is what
provides us with beautiful sunset colors on the clouds and in the
sky. A cubic inch of air normally has about 100,000 solid particles. The
air over the mid-Pacific has about 15,000, and the air above large
cities has 5 million particles per square inch.
There are other things in the air also: salt from the
ocean, pollen from plants, floating microbes, and ash from meteors which
burned upon hitting our atmosphere. There is also water vapor in the
atmosphere—and that vapor is vitally important; without it we would
quickly perish! It is part of the water cycle. But more on that in
the next section of this chapter.
Because air has weight, we have barometric pressure,
wind movement; and air resistance. The weight of all the air in the
world is about 5 quadrillion tons (That is a 5 with 15 zeros after it).
The weight of the air in a pint [.47 l] jar is about that of a small
capsule or an aspirin tablet. The greatest air pressure is found at the
earth's surface, where it averages about 15 pounds [6.8 km] pressing
down on every square inch [2.54 sq. cm]. The amount of air pressing down
on your shoulders is about 1 ton (1 short ton is 2,000 lbs. [907 km]).
Fortunately, you do not feel this weight because it is pressing on you
from all sides.
Without air, we could not have weather, and without
weather conditions there could be no rain. The
sun causes air to move by heating it. The warm air rises upward into the
colder areas above it—and clouds form. Sideways pushing and shoving of
the warm and cold air against one another causes more turbulence. But
what causes rain? We will consider that shortly.
Did you know that there are "air tides" as
well as ocean tides? Movements of the earth in relation to the moon and
sun cause ocean tides, but the gravity from the moon and sun causes air
tides also. This means that plants and people weigh a little less when
the moon is overhead.
What can be slower than air? Actually, few things are
faster! Although air may appear to move slowly most of the time, the air
molecules within it travel at extremely rapid speeds. The warmer the
air, the faster the molecules move. At freezing temperature they are
really "slow"—only moving at about 1,085 miles [1,746 km] an
hour! That is 1 1/2 times faster than the speed of sound at freezing
temperatures.
The exosphere is the highest layer of air above us and
starts at about 300 miles [482.7 km] up. There is hardly any air at that
height. Below that is the ionosphere,' which is 50 to 300 miles
[80.4482.7 km] above the earth. Electrically-charged ions found in this
part of the atmosphere protect us from solar winds and other radiation
entering from outer space. The beautiful aurora borealis, or northern
lights, glows in this region. The bottom of the ionosphere bounces radio
waves back to earth. Without the ionosphere, most radio
communications would be virtually impossible. The ionosphere is
important for its shielding effect from solar rays and meteors. Without
the atmosphere the thousands of meteors which arrive regularly would
strike the earth, destroying animal life and vegetation.
Below the ionosphere is the very important
stratosphere, which extends from about 7 miles to about 50 miles [11.26
to 80.4 km] above us. This is where the ozone layer is found. Without
that blanket of ozone, ultraviolet rays from the sun would quickly
destroy all life on earth. This is also the highest warming layer of
the atmosphere. As the sun's rays strike the ozone, it warms it. The
ozone layer helps warm the entire planet. It is about 12 to 21 miles
[19.3 to 33.8 km] up, and the warm layer is just above it. Below the
ozone layer, the stratosphere is cold (about -67°F [-55°] over the
U.S.), but without the ozone layer it would be far colder! The upper
stratosphere—in the warm layer about 30 miles [48 km] above the
ground,—the temperature is about 30°F [-1 °C].
The troposphere is of extreme importance, for this
is where the clouds are,—and where our rain comes from. This
region extends from the surface up to about 7 miles [11.26 km], but
varies with weather conditions. Every thousand feet [3,048 dm] you go
upward through the troposphere, the temperature drops about 3-4°F. The
troposphere is the region where weather occurs; above it there are
neither clouds nor storms. Above the north and south poles, it ends
about 5 miles [8 km] up; above the equator, it ends about 10 miles [16
km] above the earth's surface.
Air helps to make soil
because it contains oxygen, carbon dioxide, nitrogen, and moisture. The
oxygen, carbon dioxide, and water combine with the chemical elements in
the rocks. Along with plant, wind, and water action, this causes the
rocks to decay and break down into small particles.
Without air, plants would quickly die. Air is absorbed
and used throughout the plant. Without air in the soil a plant cannot
survive. Even the Florida cypress (one of only two trees in the
world which can have its roots permanently submerged) grows
"knobs" which stick above the surface of the swamp in order to
take in air.
Human beings would also die without that air.
All the cells in our bodies must have oxygen. They use it to change food
into energy. When you breathe, air enters your lungs. The blood stream
takes oxygen from the air in the lungs and carries it to all parts of
your body. Fresh air also makes us feel more comfortable, for it removes
the warm, damp blanket of air next to our skin. People who work in the
open air, or who know to keep their houses properly ventilated are much
healthier and live longer.
Fresh air also has negative ions,
which are important in the maintenance of good health.
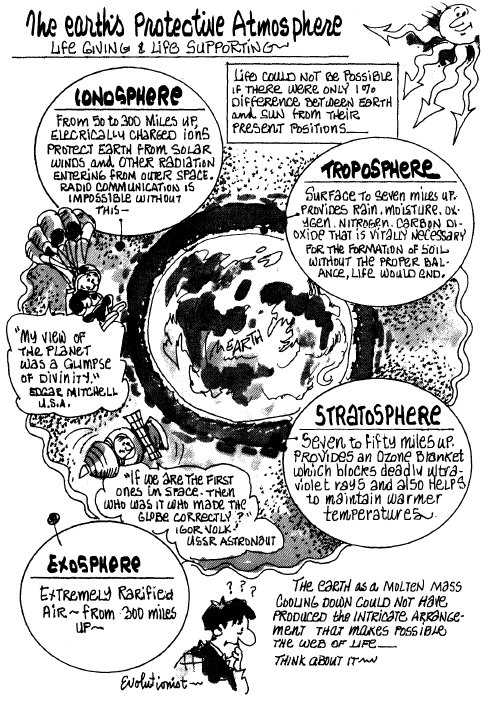
2 - WATER
Another marvelous substance is water which, when pure,
is also colorless, odorless, and tasteless. There is a lot of rock and
other material beneath our feet, but covering the surface of the planet
there is more water than anything else. Seventy percent of earth's
surface is water. Without it, nothing could live. Your body is about
two-thirds water.
There is a million million gallons of water in a cubic
mile of ocean (that is 1 with 12 zeros after it). Of the 326 million
cubic miles [524,631,800 c km] of water on earth, much of it (97
percent) is in the oceans, but there are also large amounts beneath our
feet. The upper half-mile [.8 km] of the earth's crust contains about
3,000 times as much water as all the rivers of earth. Only about 3
percent of the earth's water is fresh. About three-fourths of that fresh
water is frozen in glaciers and icecaps. There is as much frozen water
as flows in all the rivers in 1,000 years.
We can be thankful that so much water is frozen!
If it were to melt, all the seaports of the world would be below the
ocean's surface, and much of the continental coastal areas would be lost
to us also.
All living things contain lots of water. It is truly
the element of life. Your body is about 65
percent water—the same as a mouse. An elephant and an ear of corn is
about 70 percent; a chicken is 75 percent water; a potato, earthworm,
and pineapple are 80 percent; a tomato is 95 percent; a watermelon about
97 percent.
You can live a month without food, but only a week
without water. A person that loses more than 20 percent of his
normal water content becomes over-dehydrated and dies a painful death.
Each of us must take in about 2 1/2 quarts [2.4 l] of water each day in
water and food. On the average, a person takes in about 16,000 gallons
[605 hl] of water during his lifetime.
Plants, animals, and people must have a daily inflow
of nutrients. Water dissolves those nutrients so they can be carried
throughout the body in the blood stream, taken through cell walls,
and utilized by the body. The chemical reactions can only take place
in a fluid environment. We are here briefly describing processes
which are so utterly complex that mankind still has only the barest
understanding of them.
Water is needed to grow plants.
It requires 115 gallons [435 l] of water to grow enough wheat to bake a
loaf of bread. To produce 1 pound [3.7853 l) of potatoes takes 500
pounds [1,892.6 l] of water. About 41 percent of all water used in the
United States is for irrigation.
A larger amount, 52 percent, is used to keep the
factories going. Without water much of the manufacturing would stop.
It takes 65,000 gallons [2,460 hl] to make a ton [.9072 mt] of steel; 10
gallons [37.85 l] to refine a gallon [3.753 l] of gasoline; 250 tons
[226.8 mt] to produce a ton [.9072 mt] of paper. In industry, it is
especially used to clean, liquidize, but, most of all, to cool.
Without water mankind could accomplish little, much
less survive long. Yet it is all based on the water cycle. Water
evaporates from oceans, lakes, and rivers. Taken up into the air, it
falls as fairly pure water in the form of rain or snow. About 85 percent
of the water vapor in the air comes from the oceans. Plants also add
moisture to the air. After water is drawn up from the ground through the
roots, it passes up to the leaves where it exits as vapor. A typical
tree gives off about 70 gallons [265 l] of water a day, and an acre
[.4047 ha] of corn gives off about 4,000 gallons [151 hl] a day. This
continual drawing of water from the roots up through the stems, trunk,
and through the leaves gives torgor (stiffness) to the plants. Without
it, they would wilt, become flabby and die.
The oxygen and water given off by plants is part of
the reason why you feel more refreshed near plants than in a
desert or on a city street.
Water can be a solid, a liquid, or a gas.
No other substance appears in these three forms within the earth's
normal range of temperature.
Nearly every substance in the world expands as it
warms and contracts as it cools. But water is different: As it cools, it
continues to contract, and then, a few degrees before it freezes at
32°F [0°C],—it begins expanding. As it continues to cool, it
continues to expand. For this reason, ice is lighter in weight than an
equal amount of water. So the ice floats on water, instead of
sinking into it—and filling all the lakes and rivers with solid ice in
the winter. Because ice expands, the ice sheet on the surface of a
pond pushes sideways and lock against the banks on either side.
Below it, the water continues to remain liquid and the ice insulates the
water from becoming too cold and freezing also. If it were not for this
cooling expansion factor, no plants, fish, frogs, or any other wildlife
could survive a winter in rivers and lakes where freezing occurs.
It is a miracle that water is liquid at livable
temperatures. Other substances (such as H2Te,
H2Se, and H2S) which are similar to water (H20),
are gases at normal temperature, and do not change into water until the
temperature falls to -148° to -130°F [-100°C to -90°C]! As their
formulas show, they are very similar to water, each having two atoms of
hydrogen, but, instead of an atom of oxygen, they have an atom of
tellurium, selenium, or sulfur. If water was like them, there would
only be steam; no water, no water vapor, no clouds, no snow, and no ice.
Still another amazing quality of water is the fact
that, between the time it begins to boil and when it turns to steam,—it
stores so much energy as it is heated. When water reaches 212°F
[100°C], it does not immediately turn to steam, but instead there is a
pause, during which the water absorbs additional heat without any rise
in temperature. This heat is called latent heat. More than five times as
much heat is required to turn boiling water into steam as to bring
freezing water to a boil. Thus, steam holds a great amount of latent
heat energy. Because of that fact, steam can be
used to operate machinery.
Water vapor also has a tremendous amount of latent
heat energy. This energy is released when the
vapor cools, condenses, and falls as rain. The high latent heat of water
is related to its remarkable heat capacity. Heat capacity is the
ability of a substance to absorb and hold heat without itself becoming
warmer. Water can do this better than any other substance in the
world, except ammonia!
For example: If three solid substances (gold, ice
[frozen water], and iron) were placed at the temperature of absolute
zero, which is -460°F [273.3°C; 0°K]. (Absolute zero is the
theoretical temperature where a substance contains no heat of any
kind.), and then all three substances were heated, making sure that all
three were receiving (absorbing) the same amount of heat,—when that
point was reached where the gold melted at 2016°F (-1138°C],—the ice
would still be -300°F [-184.4°C]! If additional heat were equally
applied to the ice and iron, when the iron began to melt at 2370°F
(-1334°C], the ice would finally have reached 32°F [-0°C]!
Another example: take two cooking pots and put nothing
in the first (make sure it is a worthless pot!) and fill the second with
water, set both on two fires on the stove. Very quickly, the second will
get extremely hot and may turn red. At the same time, the water in the
second pot will only be starting to get warm! It had been absorbing
heat energy without itself changing much in temperature.
This ability of water to absorb heat or lose heat
without itself hardly changing temperature is an amazing quality. It is
for this reason that the oceans can store large amount of heat and
keep the planet warmer—without that water turning to steam. Conversely,
the water can give up a lot of heat before it turns to ice. For the
same reason, fish and plants can over-winter in lakes, ponds, and rivers
without freezing, and they can go through the summer without the water
boiling them to death!
Water has powerful dissolving ability.
It can dissolve almost any substance, including some of the hardest
rocks. It also dissolves the nutrients that plants and animals need for
nourishment. Dissolving the nutrients in soil, it carries them to plant
roots, and thence up through the plant to cells within the plants. It
also dissolves the food that animals and people eat. Within the body, it
carries those nutrients to each cell, and then carries off wastes.
This solvent quality enables you to wash things with
water. How would you like to take a bath in turpentine, kerosene, paint
thinner, or cleaning solvent? Water cleans best
and does it without injury.
Capillary action is the ability of a liquid to climb
up a surface against the pull of gravity.
Because of this, water is drawn up from the roots into the tops of trees
hundreds of feet in the air. The capillarity of water helps pull it
through the soil, through plants,—and through body tissues as well.
Surface tension is the ability of a substance to stick
to itself and pull itself together. Water has one of the highest
surface tensions of any substance. Because of this, water forms into
drops; it is actually clinging together! Water molecules cling together
so tightly that insects can walk on it. This tension is also a sticking
factor. It makes water able to stick to things—and wet them. In
doing this, it can dissolve substances and then transport them to
another location.
3 - SOIL
The ground beneath your feet has a lot more mysteries
and marvels to it than you might think. In
chapter 5 (Origin of the Earth), we learned that there is a thick
layer of granite beneath all the continents. This granite gives rigidity
to the continental masses, and is the foundation upon which rests the
sedimentary strata, laid down by the Flood. This granite also provides a
base on top of which are underground river channels, various pockets of
minerals, petroleum, etc. Still farther up is to be found the soil which
is close to the surface. Air, water, ice,
roots, flood, and glaciers all work to crumble the rocks near the
surface. Plant and animal remains, and body wastes, add to the mixture,
and soil is the result.
When plants die, they decay and form humus, an organic
material that makes the soil more fertile. Animal remains add to the
humus. Bacteria in the soil help the plants decay. Animals that burrow
in the soil help mix it.
An extremely valuable creature is the earthworm. It
swallows soil as it burrows, chews it up, and excretes it again. The
result is a finely pulverized soil. Earthworms feed on dead plant
material in the soil. The worms help break down the humus—the decaying
matter—in the soil. The necessary air for plant growth enters the soil
through the burrows made by the earthworms.
The topsoil is the best soil for growing plants. It is
seldom more than a foot deep [30.5 cm]. below is the subsoil, which may
be 2-3 feet ]61-91 cm] deep. This is not as rich, for the earthworms and
microbes have not worked it over, and it lacks the humus.
The ideal soil is structured so that each grain is
not entirely separate, but sticks together with others in small crumbs.
Humus is valuable in helping the soil stick together in this way. A good
soil texture is one in which particles are not too small (clay) or too
large (sand, pebbles, or small rocks). The best soils will be a mixture
of sand, clay, or silt without too much of either, plus a good amount of
humus.
There are small creatures, bacteria (also called microzyma)
which live in the soil and help condition it.
As the evening cools, dew forms on the plants and
ground and waters the earth. Plants reach
their roots down into the ground and tap underground water. But the
earth has been damaged. The aerial and underground watering system was
partially deranged at the time of the Flood. Another problem was
deposition by flood waters of sections of clay, sand, exposed rock,
gravel, and calcite, iron, selenium and other beds. Soils may lack
calcium or have too much (and thus be too acid or alkali).
When too much rain falls, erosion results as soil is
carried off. Rain also leaches the soil, taking nutrients downward into
the ground. But while the top layer is
leached by rainwater, minerals in the rock beneath
it can be reached by plant and tree roots, which draw up more nutrients.
In addition, humus can be built up by falling leaves and stalks, and
by man as he works with the soil.
The result is garden plants containing the nutrients
needed for life. We plant, tend, harvest, and eat the plants and
obtain the vitamins, minerals, carbohydrates, and proteins needed for
the sustenance of life. We drink the water from the skies, and bathe
our bodies in it. The sunlight falls upon us and deepens our health.
Amid all the work, we grow stronger. It is all part of a good plan
by One who looked upon the world when it was first made and declared,
"It is good."
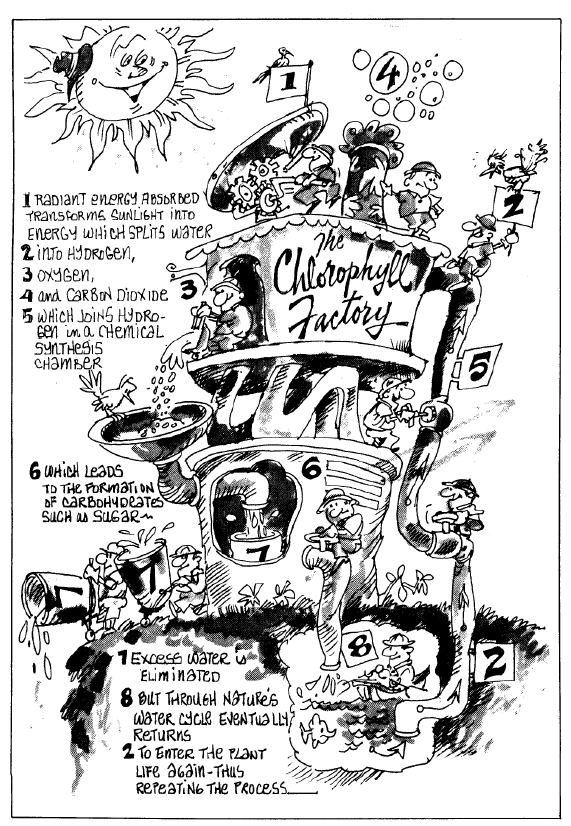
4 - CONCLUSION
In air, water, and soil we see basic provisions for
life on our planet. It is true that the Flood
damaged the soil and inundated much of the world with oceans. But in and
through it all a careful plan is revealed, so that plants, animals, and
man can live in our world. Yes, it takes work, but work was given to
mankind as a blessing.
The promise has been given that someday the earth will
be restored to the Edenic beauty it had before the Flood. But even now
we have many good things. This world was designed for plants, animals,
and people to live. The arrangement did not come about by chance. Too
many factors are involved, and if even one was missing, life could not
exist here.
Recent scientific studies have disclosed that if the
sun had been just a little closer or farther away from our planet, no
life could survive. Scientists have discovered that if the Earth was
only one percent closer to the sun, or one percent farther away from
it,—we would all quickly perish!
If the earth's magnetic outer barrier did not exist,
solar winds and other radiation would render it impossible for anyone to
live. If the oceans did not exist after the Flood, not enough rainfall
could fall on the continents. Without broad oceans there would not be
enough oxygen, since small ocean plants called plankton make most of it.
Without the ability of water to absorb and retain heat—plus the great
ocean currents—much of the world's continental areas would be too hot
or cold to live in. We cannot drink seawater, and without winds and
storms we could not have rain, rivers, lakes, and countless other
blessings.
Yes, our world was designed for people, animals, and
plants. A molten mass cooling down (such as is
theorized by evolutionists as earth's beginnings), could not have
produced the intricate arrangement that makes possible the web of life
we now see about us on planet Earth.
THE VIEW FROM SPACE
Western astronauts and Soviet cosmonauts have had an
opportunity to see the earth from outer space. All who have done so have
been awed by the sight. Here are a few selected quotations from men who
have had an unusual opportunity to realize how wonderfully designed is
our planet.
"Space is so close: It took only eight minutes
to get there and twenty to get back."—Wubbo
Ockels, in Kevin W. Kelley, The Home Planet (1988) [Netherlands].
"There is a clarity, a brilliance to space that
simply doesn't exist on earth, even on a cloudless summer's day in the
Rockies, and nowhere else can you realize so fully the majesty of our
Earth and be so awed at the thought that it's only one of untold
thousands of planets."—Gus Grissom,
Gemini: A Personal Account of Man's Venture into Space (19678) (USA].
"The sun truly 'comes up like thunder,' and it
sets just as fast. Each sunrise and sunset lasts only a few seconds.
But in that time you see at least eight different bands of color come
and go, from a brilliant red to the brightest and deepest blue. And
you see sixteen sunrises and sixteen sunsets every day you're in
space. No sunrise or sunset is ever the same."—Joseph
Allen, "Joe's Odyssey," in Omni, June 1983, p. 63 [USA].
"We entered into shadow. Contact with Moscow
was gone. Japan floated by beneath us and I could clearly see its
cities ablaze with lights. We left Japan behind to face the dark
emptiness of the Pacific Ocean. No moon. Only stars, bright and far
away. I gripped the handle like a man hanging onto a streetcar. Very
slowly, agonizingly, half an hour passed, and with that, dawn on
Earth.
"First, a slim greenish-blue line on the
farthest horizon turning within a couple of minutes into a rainbow
that hugged the Earth and in turn exploded into a golden sun. You're
out of your mind, I told myself, hanging onto a ship in space, and to
your life, and getting ready to admire a sunrise. "—Valeri
Ryumin, 176 Days in Space: A Russian Cosmonaut's Private Diary — And
an Incredible Human Document, p. 15 [USSR].
"Firefly meteorites blazed against a dark
background, and sometimes the lightning was frighteningly brilliant.
Like a boy, I gazed open-mouthed at the fireworks, and suddenly,
before my eyes, something magical occurred. A greenish radiance poured
from Earth directly up to the station, a radiance resembling gigantic
phosphorescent organ pipes, whose ends were glowing crimson, and
overlapped by waves of swirling green mist.
" 'Consider yourself very lucky, Vladimir,' I
said to myself, 'to have watched the northern lights.' "—Vladimir
Remek, in Kevin Kelley, The Home Planet (1988), [Czechoslovakia].
"I shuddered when I saw a crimson flame through
the porthole instead of the usual starry sky at the night horizon of
the planet. Vast pillars of light were bursting into the sky, melting
into it, and flooding over with all the colors of the rainbow. An area
of red luminescence merged smoothly into the black of the cosmos. The
intense and dynamic changes in the colors and forms of the pillars and
garlands made me think of visual music. Finally, we saw that we had
entered directly into the aurora borealis.—
"Aleksandr Ivanchenkov, in Kevin Kelley, The Home Planet (1988),
[USSR].
"The Earth reminded us of a Christmas tree
ornament hanging in the blackness of space. As we got farther and
farther away it diminished in size. Finally it shrank to the size of a
marble, the most beautiful marble you can imagine. That beautiful,
warm, living object looked so fragile, so delicate, that if you
touched it with a finger it would crumble and fall apart. Seeing this
has to change a man, has to make a man appreciate the creation of God
and the love of God."—James B. Irwin,
in J.B. Irwin and W. A. Emerson, Jr., To Rule the Night (1982) [USA].
"Suddenly from behind the rim of the moon, in
long, slow-motion moments of immense majesty, there emerges a
sparkling blue and white jewel, a light, delicate sky-blue sphere
laced with slowly swirling veils of white, rising gradually like a
small pearl in a thick sea of black mystery. —It takes more than a
moment to really realize this is Earth; this is home!"—Edgar
Mitchell, Noetic Scientific Brochure (1982) [USA].
"On the way back [from the moon] we had an EVA
[extra-vehicular activity, or spacewalk] I had a chance to look around
while I was outside and Earth was off to the right, 180,000 miles
away, a little thin sliver of blue and white like a new moon
surrounded by this blackness of space. Back over my left shoulder was
almost a full moon.
"I didn't feel like I was a participant. It was
like sitting in the last row of the balcony, looking down at all of
that play going on down there . . I had that insignificant feeling of
the immensity of this, God's creation."—Charles
Duke, Jr., in Kevin Kelley, The Home Planet (1988) [USA].
"Several days after looking at the Earth a
childish thought occurred to me—that we the cosmonauts are being
deceived. If we are the first ones in space, then who was it who made
the globe correctly? Then this thought was replaced by pride in the
human capacity to see with our mind."—Igor
Volk, in Kevin Kelley, The Home Planet (1988) [USSR].
"You see layers as you look down. you see
clouds towering up. You see their shadows on the sunlit plains, and
you see a ship's wake in the Indian Ocean and brush fires in Africa
and a lightning storm walking its way across Australia. You see the
reds and the pinks of the Australian desert, and it's just like a
stereoscopic view of all nature, except you're a hundred ninety miles
up. "—Joseph Allen, "Joe's
Odyssey," in Omni, June 1983, p. 63 [USA].
"Myriad small ponds and streams would reflect
the full glare of the sun for one or two seconds, then fade away as a
new set of water surfaces came into the reflecting position. The
effect was as if the land were covered with sparkling jewels."—Karl
Henize, in Kevin Kelley, The Home Planet (1988) [USA],
"The Pacific. You don't comprehend it by
looking at a globe, but when you're traveling at four miles a second
and it still takes you twenty-five minutes to cross it, you know its
big."—Paul Weitz, quoted in Henry F.S.
Cooper, A House in Space (1976) [USA].
"Although the ocean's surface seems at first to
be completely homogeneous, after half a month we began to
differentiate various seas and even different parts of oceans by their
characteristic shades.
"We were astonished to discover that, during an
flight, you have to learn anew not only to look, but also to see. At
first the finest nuances of color elude you, but gradually your vision
sharpens and your color perception becomes richer, and the planet
spreads out before you with all its indescribable beauty. "—Wadimir
Lyakhov, quoted in J. E. and A. R. Oberg, Pioneering Space (1986)
[USSR].
"We were able to see the plankton blooms
resulting from the upwelling off the coast of Chile. The plankton
itself extended along the coastline and had some long tenuous arms
reaching out to sea. The arms or lines of plankton were pushed around
in a random direction, fairly well-defined yet somewhat weak in color,
in contrast with the dark blue ocean. The fishing ought to be good
down there."—Edward Gibson, quoted in
Henry F.S. Cooper, A House in Space (1976) [USA]..
"As we were flying over the Mozambique Channel,
which separates the island of Madagascar from the continent of Africa,
we could clearly see the transverse sand bars at its bottom. It was
just like a brook one waded in childhood. "—Lev
Demin, in Kevin Kelley, The Home Planet (1988) [USSR].
"The first day or so we all pointed to our
countries. The third or fourth day we were pointing to our continents.
By the fifth day we were aware of only one Earth." —Sultan
Bin Salman al-Suad, in Kevin Kelley, The Home Planet (1988) [Saudi
Arabia].
"We had various kinds of tape-recorded concerts
and popular music. But by the end of the flight what we listened to
most was Russian folk songs. We also had recordings of nature sounds:
thunder, rain, the singing of birds. We switched them on most
frequently of all, and we never grew tired of them. It was as if they
returned us to Earth. "—Anatoli
Berezovoy, in V. Gor'koy and N. Kon'kov, Cosmonaut Berezovoy's Memoirs
on 211-Day Spaceflight (1983) [USSR].
"A strange feeling of complete, almost solemn
contentment suddenly overcame me when the descent module landed,
rocked, and stilled. The weather was foul, but I smelled Earth,
unspeakably sweet and intoxicating. And wind. Now utterly delightful;
wind after long days in space. "—Andriyan
Nicolayev, in Kevin Kelley, The Home Planet (1988) [USSR].
MORE WONDERS OF DESIGN
MATHEMATICS OF A SWIFTLET'S CLICKS
—Swiftlets are small birds that live in southeastern Asia and
Australia. They make their nests far back in dark caves. It is not
difficult for an owl to fly through the woods at night, for a small
amount of light is always present and owls have very large eyes. But the
situation is far different for a swiftlet. There is no light in caves!
And swiftlets have small eyes! How then is this little creature able to
find its way through a cave, without running into the walls? Yet he does
it.
Designed with fast-flying wings, such as swallows and
swifts have, the swiftlet flies at high speed into its cave. Somehow it
knows which cave to fly into. But, once inside, there is no glimmer of
light to guide it. Yet rapidly and unerringly, it flies directly to one
tiny nest. Arriving there, it is confronted with hundreds of nests which
look exactly the same. How can it know which one is its own?
Nevertheless, flying at top speed, the bird flies across even the
largest cavern in only a few seconds-and then lands at the correct nest.
Part of the mystery is solved when we consider that
the swiftlet has been given a type of radar (sonar) system. But this discovery
only produces more mysteries. As the little bird enters the cave, it
begins making a series of high-pitched clicks. The little bird has the
ability to vary the frequency of the sounds; and, as it approaches the
wall, it increases the number of clicks per second until they are
emitted at about the rate of about 20 per second. The time required for
the clicks to bounce off the wall and return reveals both the distance
to the wall and its contours.
Scientists tried to figure out why the clicks vary in
frequency as the bird gets closer to the wall. After applying some
complicated mathematics, they discovered that the tiny bird —with a
brain an eighth as large as your little finger —does this in order to
hear the return echo! The problem is that the click must be so short and
so exactly spaced apart, that its echo is heard by the ear of the bird
—before the next click is made. Otherwise the next click will drown
the sound of the returning echo.
FOG-DRINKING BEETLE —How
can a wingless beetle, living in a desert, get enough water? This
one does it by drinking fog.
Onymacris unguicularis is the name of a little beetle
that lives in the rainless wilderness of the Namib Desert, close to the
southwestern coast of Africa. This flightless beetle spends most of its
time underground in the sand dunes, where temperatures remain fairly
constant. But when thirsty, it emerges from its little burrow and looks
about. There is no water anywhere; rain comes only once in several
years. The little fellow is not discouraged, but climbs to the crest of
a sand dune, faces the breeze, and waits. Gradually fog condenses on its
body. It just so happens that this beetle is born with several grooves
on its face. Some of the water trickles down the grooves into the
beetle's mouth. Happily, the little fellow goes searching for dry food
and then returns to its burrow for a nap.
ELECTRICAL IMPULSES OF KNIFE FISH
—The Amazon knife fish is a strange looking creature. It has no
fins on the side, top, or tail; all its fins are beneath it —in one
long, single wave of fin from front to back! Indeed, this eight-inch
fish has no tail at all. The fish looks somewhat like a sideways
butter-knife, which narrows to a spear point at its hind end.
Its one, long ribbon-like fin undulates from one end
to the other —something like millipede legs which move it through the
water. As it travels, it can quickly go into reverse gear and swim
backwards with that fin.
But the most unusual feature of this little fish is
its lateral line. This horizontal line of cells on its side is an
electrical generating plant, producing impulses which are sent out into
the water to both one side and the other. These impulses bounce off
objects and quickly return where they are sensed by other receptor cells
in its skin. The voltage of these cells is low, only about 3 to 10 volts
of direct current. Yet the frequency of the impulses is high-about 300 a
second. As these impulses go outward, they create an electrical
sending/receiving field of signals, which tell the fish what is around
it —in front, to the side, and even to the rear.
But imagine the problems which ought to occur when two
knife fish come near each other! Both fish are sending out signals, and
the resulting incoming confusion of patterns would be expected to
"blind" both fish. But, no, the Designer gave these fish the
ability to change wavelengths! As soon as two knife fish draw near to
one another, they immediately stop transmitting impulses for a couple
moments, and then both switch them back on —but this time on different
frequencies to each other!
UNDERGROUND FLOWERS —We
all know that flowers never grow underground; but here are two that do:
There are two Australian species of orchid which, not
only produce flowers under the earth's surface, —but the entire plants
are there also! The only exception is a tiny cluster of capsules which
is occasionally pushed up to disperse the dust-like seeds.
How can these plants live underground? Both species
feed on decaying plant material in the soil, breaking it down with the
aid of fungi. They do all their growing and blooming beneath the top of
the soil. Their flowers are regular orchid flowers!
The first, Rhizanthella gardneri, was
discovered by accident in 1928 by J. Trott, a farmer who was plowing a
field near Corrigin, western Australia. The second, Cryptanthemis
slateri, was found by E. Slater in 1931 at Alum Mount in New South
Wales. The little plants keep so well hidden that few have ever been
found since then.
KNOWING WHERE TO JUMP
—Gobies are small fish which, during low tides, like to swim in rock
pools on the edge of the ocean. One species, the Bathygobius, enjoys
jumping from one tidal pool, over rocks exposed above the water, into
another rock pool on the other side. Researchers finally became
intrigued by this habit and decided to investigate.
They discovered that this little fellow always jumps
just the right amount, at the right place, and in the right direction
—without ever landing on rock! How can this fish know where to leap
out of the water, and in what direction? It cannot see from one rock
pool to the next. Surely it does not have the locations and shapes of
all the rock pools pre-memorized in its tiny head! Although much of the
area around a pool is exposed rock, with no nearby pools beyond it, yet
the Goby always jumps at exactly just the right place. The scientists
have guessed that, perhaps, when the tide earlier came in and covered
all the rocks, the fish swam around and memorized all the bumps and
hollows on the rock, and thus later knows where to do its jumping. But,
if that were true, then the mystery would only deepen even more. How
could this very small fish have enough wisdom to go about in advance and
learn all that?
VARIETIES OF ROSES —In chapter
13 (Natural Selection) we discuss the wide range of possibilities
to which each natural species can be bred. Because of this, large
numbers of subspecies can be developed. The making of new subspecies is
not evolution.
An example of this would be the rose. More than 8,000
varieties of rose have been developed for garden cultivation, yet all of
them are descended from only a few wild forms. Although roses have been
cultivated by the Persians, Greeks, Romans, and Europeans, there were
only four or five rose types by the end of the 18th century. This
included the dog rose, musk rose, and red Provins rose.
Modern varieties, such as the hybrid tea rose
(single-flowered) and floribundas (cluster-flowered), began to be bred
only around 1900, after the European species were crossed with
cultivated oriental Chinese imports.
MIGRATING LOBSTERS —Spiny
lobsters live and spawn near coral reefs of the Bahamas and the
Florida coast. But each fall, the lobsters know that it is time to
leave. Storms occur throughout the year; yet, for some unknown reason,
at the time that one of the autumn storms stirs the waters, the lobsters
quickly know that migration time has come. Within a few hours they
gather in large groups.
Then they form into long, single-file lines and begin
marching out into the ocean. They always know to move straight out, and
not sideways. As they travel on the sand, each lobster touches his long
antennae on the rear of the one in front of him. There is no hesitation
about these marches; the creatures gather and immediately depart. As
they go, they travel surprisingly fast, yet maintain their alertness.
They can never know when their main enemy, the trigger fish, or another
predator may suddenly dart down through the clear waters. Indeed, the
lobsters are easy to see, for the tropical sands beneath them are often
white.
When a trigger fish does arrive, the lobsters
instantly go into action. They form into circles, with their pincers
held outward and upward in a menacing gesture. When the trigger fish,
decides it is not worth getting pinched and leaves, the spiny lobsters
reform into a line and continue their march. Finally, they reach a lower
level and remain there throughout the winter. Since less food is
available during the winter months, at these lower levels the colder
water temperature helps slow their metabolism and they go into
semi-hibernation until spring returns. Then they march in lines back to
their summer feeding grounds.
Who put all this understanding into the minds of the
little lobsters? Could you train a lobster to do all that?
POP GOES THE MOSS
—The various sphagnum mosses (the kind you purchase at garden
supply stores as mulch) grow in peat bogs. These mosses have a special
way of ejecting their seeds.
In the final stage of ripening, the spore capsules
shrink to about a quarter of their original size, compressing the air
inside, and reshape into tiny gun barrels, each with its own airtight
cap. Each barrel is very small-about 0.1 inch in length.
Then the cap breaks under pressure, and the trapped
air escapes with an audible pop, firing the packet of spores as far as 7
feet. How could this tiny plant devise a battery of natural air guns to
disperse its dust-like spores?
Evolutionists glibly tell us it all happened "by
accident." But, first, it could not happen by accident. Second, it
could not even happen by human design. It would be impossible for a
person to get a plant to do the things these little mosses regularly do
in the process of preparing their seeds, packing them in for firing, and
then shooting them off.
SPIDER MAKES HIS DOOR
—Although only an inch long, the female trap-door spider makes
excellent doors and latches. After digging a burrow six inches deep into
soft ground, she lines the walls with silk, and then builds the front
door.
This is a circular lid about three-quarters of an inch
across. A silken hinge is placed on one end, and gravel on the bottom.
In this way, as soon as the lid is pulled over, it falls shut by its own
weight. The top part of the door exactly matches the surroundings; and,
because it just happens to have a carefully made beveled edge, the door
cannot by the closest inspection be seen when closed.
Throughout the day, the door remains shut, and the
little spider inside is well-protected from enemies. When evening comes,
the door is lifted and the little creature peers out to see if it is
dark enough to begin the night's work.
With the door open wide, the spider sits there, with
two front feet sticking out, awaiting passersby. When an insect happens
by, the door is shut and lunch is served.
Sometimes the spider locks the door. This is
especially done during molting time, when the door is tied down with
ropes of silk. The males build similar tunnels.
FAST-GROWING TREES —It is
always a marvel how a tiny seed can grow into a mighty tree. But,
although it takes time for a tree to grow, some trees grow very rapidly.
The fastest-growing tree in the world is the AIbizzia
falcafa, a tropical tree in the pea family. Scientists in Malaysia
decided to measure how fast one could grow, and found it reached 35.2
feet in 13 months. Another in the same region grew 100 feet in five
years. The Australian eucalyptus is also a speedy grower. One specimen
attained 150 feet in 15 years.
BABY GLUE GUNS —Ants have
discovered that babies make good glue guns.
The green tree ants of Australia make their homes out
of living leaves. Several workers hold two leaves together, while others
climb up the tree trunk carrying their children (the little grubs which
will later change into adult ants). Arriving at the construction site,
these ants give their babies a squeeze, and then point them toward the
leaves. Back and forth they swing their babies across the junction of
the leaves, and out of the baby comes a glue-like silk which spot-welds
the leaves together. It looks as if a white, silken network is holding
the leaves together. When the building project is finished, the ants
move into their new home. Perhaps they thank their young for providing
the nails to hold the house together.
MILKING THE TREES —That
is what they do in Venezuela: milk trees.
The South American milk tree (Brosimum utile) belongs
to the fig family and produces a sap that looks, tastes, and is used
just like cow's milk. Farmers go out and collect it. The trees are easy
to care for; it is not necessary to chase after strays, string barb
wire, round up the herd and put them into barns at night, or teach the
young to drink out of pails.
RUNNING ON WATER —How can
a skimmer —the little rove beetles which glide effortlessly over a
water pond —run across the surface of the water?
It is now known that they are pulled by the surface
tension of the water ahead of them. But how can this be, for is there
not just as much surface tension in the water behind them? No, there is
not.
These little skimmers can only travel as fast as they
do —because they lower the surface tension at the rear of their bodies
in a very special manner. There is a small gland at the back end of
their abdomens. A tiny amount of fluid from that gland is placed on the
water as they run along. This fluid lowers the water's surface tension!
But the surface tension ahead of them remains high —and it is an
obscure law of physics that this difference tends to pull them forward!
Seriously now: What self-respecting beetle would be
able to figure out the complex chemical formula for that fluid, much
less planning how to restructure its body in order to manufacture it in
the gland it is produced in? How would he know enough about physics to
understand, in the first place, what he was trying to do?
Or could you, with your large brain, restructure your
body? There is hardly a boy in the land who would not like to have the
muscles and endurance of the tiger, but he cannot get it.
If we cannot change our bodies, why should anyone
imagine that animals can do it?
MORE ABOUT CLOWNFISH—In
chapter 24, we discuss the astonishing activities of the clownfish,
which lives amid the stinging tentacles of the anemones without ever
being injured by them. Scientists have puzzled over this for years. It
has recently been discovered that the answer is that other fish have a
certain chemical in the mucus covering their bodies which, when touched
by the arm of an anemone, causes its stings to discharge. Clownfish lack
this chemical, and are thus able to live amid those tentacles, and let
the anemone defend them.
In addition, in the reefs off Australia and New
Guinea, the clownfish protects the anemone. The butterfly fish is in
that region, and —also lacking that chemical —it is able to bite off
parts of the anemone. But when it swims near, the little clownfish comes
out and attacks it, driving it away. In this way, the clownfish protects
the anemone which protects it.
FISH THAT BUILD NESTS
—Some fish are born in nests. The labyrinth family (which
include the Siamese fighting fish) are air-breathing fish. They build
nests in vegetation near the surface. Sticky bubbles are blown by the
male, who places the eggs in the nest and watches over them until they
are born, and thereafter for a time.
The stickleback fish also builds nests. The male
collects pieces of aquatic plants, and glues them together with a cement
secreted from its kidneys. Placing the plant mass in a small pit in the
sand, it then makes a burrow or tunnel inside, where the eggs are then
laid.
Other fish form depressions in the sand and remain
there to care for their young after they hatch. But no other nesting
material is used.
Nesting, whether done by birds or fish, is actually a
very complicated pattern. It is not something that a weak-minded bird or
fish could ever have thought up by itself. Yet most birds and some fish
regularly do it.
It is of interest that, even if a solitary bird had
actually stumbled upon the idea of making a nest, that bird would not
have taught it to its babies. So the pattern would have stopped right
there. Just as there is no way that the pattern could be started, there
is no way it could be passed on to the next generation. "Oh,"
someone will reply, "the information simply passed into the
genes." Not so, any good scientist will tell you that there is no
such thing as inheritance of acquired characteristics.
STICK-BEATING BIRD —No,
this isn't a stick beating a bird, but a bird beating with a stick.
The huge black palm cockatoo of northern Australia enjoys screeching
high notes and whistling low ones to its neighbors. It wants everyone to
know it is there. Yet even this is not enough to satisfy it.
To insure that no rival cockatoos enter their
territory, breeding pairs signal their ownership of a territory by
breaking off a small stick with their claws and beating it against a
hollow tree.
HEAD-DOOR FROGS —Some Mexican
tree frogs use their heads to survive. Called helmet frogs, they
have bony crests on top of their skulls. When drought begins, these
little frogs climb into tree trunks or into holes in bromeliads (plants
of the pineapple family) that grow high in trees.
Once inside, they use the tops of their heads to seal
off the entrance! Then they just sit there till rain falls again. Little
water is lost through their head, and it makes an excellent camouflage
at the doorway to their home.
MILKY WAY CAVES —The fungus-gnat
of New Zealand lives in dark caves. You can find them there by the
millions. Each of these little insects first makes a horizontal maze,
which looks something like a spider web. Then it drips down several
dozen mucus threads, which hang downward from its nest. Each of these
threads has globs of glue at several points on the thread-and those
threads glow in the dark.
Entering one of these caves and gazing upward, you
will see the steady, unblinking light of millions of stars overhead.
Some seem slightly closer, and some farther away. Everywhere you look
above you, the stars shine.
SKIN BREATHERS —Most amphibians
breathe with gills when they are larvae in the water, and later with
lungs when they become adults and live on land. But there are also
land-living, cave-dwelling, tree-climbing, and water-living species that
do not breathe through lungs or gills. Instead, they breathe through
their skin!
An example of this would be the frogs of the genus
Telmafobius. These little frogs live underwater in lakes in the high
Andes. That water is cold! Yet these frogs, having no gills or lungs,
are able to absorb oxygen from the water through their skin.
EGG PRODUCERS —Some people
wish each hen in their chicken yard would produce at least one egg a
day. But some creatures can do better than that. A single female cod can
produce six million eggs in one spawning. A female fruit fly is far too
small to do as well as the codfish, but, even so, can lay 200 eggs in a
season in batches of a hundred at a time.
Yet there are creatures which can produce far more
eggs than that. These include the corals, jellyfish, sea urchins and
mollusks. The champion is the giant clam. Once each year, for 30 or 40
years, it will shoot one thousand million eggs out into the water. This
is 1,000,000,000, or a full billion.
The largest number of young produced by any placental
mammal is that of the Microtus, a tiny meadow mouse living in North
America. This little creature can give birth to 9 babies at a time, and
produce 17 litters in a breeding season. Thus it is capable of producing
150 young each year.
MOST EXTENSIVE MINER —The
Russian mole rat is a champion burrower. In its search for
underground bulbs, roots, and tubers, it excavates long tunnels that
include resting chambers, food storage rooms, and nesting areas.
Scientists excavated one tunnel system in the former Soviet Union and
found it was 1,180 feet in length. They calculated that it took about
two months to construct.
The Russian mole rat is blind and digs with its teeth,
not with its claws. It rams its head into the soil to loosen it as it
chews out new tunnels. Every so often, it comes to the surface and makes
a mound of earth from the tunnel. The longest tunnel had 114
interconnected mounds. If that little rat can do that, just think what
you can accomplish!
CHILDREN'S CHILDREN-The
greenfly is a live-bearer insect, which means it does not lay eggs but
brings forth its babies live, as mammals do.
But the greenfly does it a little differently. During
the summer months, when there are lots of food plants in leaf, she
produces eggs within herself which are self-fertile; that is they were
never fertilized by a male. In addition, all her eggs will hatch into
females. But there is more: Each of her daughters will automatically be
fertile, so that daughter will, in turn, be able to lay fertile eggs.
MORE ON THE KANGAROO —In
another chapter, we discuss the kangaroo. But here is more
information:
After being born, the baby kangaroo journeys to its
mother's pouch and begins nursing. After about 9 months it will begin
climbing out of its mother's pouch and begin feeding. But, at times, it
will jump back in and continue taking milk. Then, at 10 months it no
longer jumps in, but remains with its mother and reaches in from time to
time to take more milk, until it is 18 months old.
There are two striking facts about this: (1) The
mother frequently has already given birth to another tiny baby which is
also in the pouch nursing, so she will have a baby and an adolescent
nursing at the same time. (2) The teat giving milk to the infant
produces different milk than the one which the older one drinks from! It
matters not which teat it is; the older one will always receive a
different composition of milk than the baby kangaroo is given. The tiny
infant has very different nutritional needs. But the question is how can
the mother vary the type of milk which is given, at the same time, to
both an adolescent and an infant kangaroo?
An example of this is the red kangaroo, which provides
milk both to a tiny joey attached in the pouch to a teat, and also to a
large joey which has left the pouch. The older one is given milk with a
33 percent higher proportion of protein and a 400 percent higher
proportion of fat.
IDENTICAL QUADRUPLETS EVERY TIME
—The female nine-banded armadillo is a common armadillo, which ranges
from the southern United States to northern Brazil. It only bears
identical quadruplets. This means that all four babies in each litter
come from one egg, which split after fertilization. So each litter is
always the same sex.
FRIGHTENING THE ENEMY
—Evolutionists tell us that creatures in the wild think through
the best ways to avoid being attacked, and then develop those features.
But, of course, this cannot be true. There is no way an animal can
change its features, or through "inheritance of acquired
characteristics,'' give them to its offspring. But the myth is adhered
to, because the obvious explanation is unwanted. The truth is that a
Master Designer provided the little creature with what it needed.
The Australian frilled lizard is about 3 feet long.
When an enemy draws near, this lizard raises a frill which normally is
flat along the back. This frill stands out in a circular disk which can
be 2 feet across. How did that frill get there? Did the lizard ''will
it" into existence? Did it tinker with its own DNA? How does it
know to use it to frighten enemies?
The lizard adds to this immense, apparent increase in
size by opening its mouth, which is bright yellow inside. By now, the
situation is surely looking worse, as far as the predator is concerned.
Then, to settle the matter once and for all, the lizard gives a terrible
hissing sound and slowly moves toward the enemy. By that time, the
troublemaker generally decides to leave.
BABY NURSERY —The eider
duck sets devotedly on her eggs without eating anything. When they
hatch, she leads them down to the pond. Entering it with her newborn
there are often many other ducklings already there that are supervised
by one or two adult females, some of which are not mothers. She leaves
her brood with them, and departs to find food. Because some of the food
is in deeper waters, she may be gone for several days. Upon her return,
she, at times, will help take care of the nursery while other mothers
leave.
The French word for "nursery" is crèche
(pronounced kresh). When animals care for their babies in nurseries,
scientists call it a "crèche." Some eider duck crèches have
been counted at over 500. If marauding gulls appear, the adult females
sound an alarm, and the young gather close about them. If the gull tries
to catch one, the adult will try to grab him by the legs and pull him
down into the water. As for the chicks, they only need protection from
these adult nursery attendants, for they are well-able to find food for
themselves.
In South America, the Patagonian cavy (which is
somewhat similar to the guinea pig) is also initially cared for in a crèche
of babies hidden in a tunnel by the rocks. One of the fathers cares for
the group till the mothers return from feeding. Upon her arrival, she
gives a call and out come about a dozen cavies. She sniffs among them,
until she finds her two, and then leads them away.
More babies are dropped off, and more mothers return
for theirs. The babies remain in the nursery tunnel, guarded by an adult
above. Adults never use the tunnel, although they initially dig it for
the nursery.
When bats return to their caves after feeding, they
must find their own within a nursery of a million or more baby bats!
Each mother flies in and lands close to her own. Then she calls for
several seconds and her baby gives an answering squeak. Formerly it was
believed that they merely nursed whatever baby they landed near. But
genetic tests established that it was their own. How they find their own
child in such an immense nursery is astounding. After nursing her own,
she flies off to another section of the large cave, hangs from the
ceiling, and sleeps for a time. Then she flies off to obtain more food
to feed her only baby.
VISION SKIN-DEEP —Some insects
can see light through their skin, even when their eyes are covered.
Experiments were done on moth and butterfly caterpillars, when their
eyes were covered. There are other insects which also have this ability.
In addition, they often have eyes in very unusual
places, as we discuss in a later chapter.
SUNGLASSES TOO —Yes, even
sunglasses existed in nature before man began using them. Seabirds, such
as gulls, terns, and skuas have built-in sunglasses. All day long they
have to search for food, as they glide above the ocean's surface.
Staring down into the waves for fish, the glint of sunlight on the waves
reflects up into the eyes. The solution is sunglasses, which they have.
The retinas of these birds contain minute droplets of
reddish oil. This has a filtering effect on light entering the eye, and
screens out much of the sun's blue light. This cuts down on the glare,
without lessening their ability to see the fish near the surface.
FLICKER'S LONG TONGUE —In
another chapter we discuss the woodpecker. Here is additional
information on his amazing tongue, and that of the flicker:
Woodpeckers like to eat beetle grubs. Cocking their
heads to one side and then another, they carefully listen for them. When
the grub is heard chewing its way through the wood —which it does most
of the time, —the bird swiftly bangs on the tree with its sharp bill,
drilling a hole as it proceeds.
Then it reaches out its enormously long tongue. How
can a tongue be four times as long as the beak, when the beak itself is
very, very long? It took special designing; accidents could never have
produced the tongue of the woodpecker.
This tongue is attached to a slender bony rod housed
in a sheath which extends back into its head, circles around the back of
its skull and then extends over its top to the front of the face. In
some woodpecker species, it also coils around the right eye socket.
Then there is the American flicker. This
woodpecker-like bird is equally amazing. The tongue is so long that,
after reaching around the back of the skull, it extends beyond the
eye-socket and into the upper beak. Here it enters the right nostril so
that the bird can only breathe through the left one. Flickers use this
tongue to extract ants and termites after drilling for them.
But a tongue is not enough. The flicker must put
something on the tongue to deal with those ants. Its saliva, wetting the
tongue, does two things: first, it makes it sticky, so the ants will
adhere to it; and, second, the saliva is alkaline, to counteract the
formic acid of ant stings.
The evolutionists will tell us that all this came
about by slow, laborious chance. But, obviously, such complicated
structures and functions could not develop by accident even once in
millions of years. Yet in the world we find six others, totally
different creatures which use this long, sticky tongue method to catch
ants: the numbat, a marsupial in Australia (which is something like a
small antelope); the aardvark in Africa; the pangolins in Asia and
Africa (which are covered with horny plates, so they resemble giant
moving fir-cones); and three very different anteaters of South America:
the gazelle-sized giant of the savannahs, the squirrel-sized pygmy which
lives in the tops of forests, and the monkey-sized tamandua which lives
in the mid-tree levels.
As usual, the evolutionists have no answer. To make
matters worse, paleontologists tell us that they can find no fossil
evidence of any antiquity to explain these matters to us. In other
words, there is no evidence that the woodpecker, flicker, anteater, and
the others evolved from anything else.
JOURNEY TO THE UNKNOWN
—Later we consider the marvel of bird migration. Here is yet another
example:
The bronze cuckoo of New Zealand (which lays its eggs
in the nest of other birds) abandons its young in the care of their
foster parents, and flies to its off-season feeding grounds, located far
away. After the babies hatch, they become strong enough to fly. But they
have never seen their parents and have no adult bird to guide them.
Added to this is the fact that, when their parents left New Zealand,
they flew to a place that no other bird in New Zealand migrates to.
So, as soon as these babies are strong enough for
vigorous flight, what do they do? Why, they fly after their parents
—and take exactly the same route. Here is the story:
The young set out each March on a 4,000-mile migration
from their parents' breeding grounds in New Zealand. They fly west to
the ocean's edge and out over it. How would you like to do that? The
Pacific is an incredibly big ocean.
With no bird to instruct or guide them, these young
birds accurately follow the path of the parent flock over a route of
1,250 miles of open sea. Arriving in northern Australia, they turn
north, fly to the ocean's edge —and start off again. Arriving in Papua
New Guinea, they head off again. This time they fly the grueling
distance to the Bismarck Archipelago.
Just one slight error in direction, and they would
die. Why? Because not one of the birds can swim.
AMAZING HOUSE OF THE TERMITE
—Termites build their homes of mud. Their homes are amazing
structures, as we will learn below. Yet those large, complicated
buildings are made by creatures which are blind. They have no
instructors to teach them, and they spend their lives laboring in the
dark. Nevertheless, they accomplish a lot.
Termites, of which there are over 2,000 species, only
feed on dead plants and animals, and have very soft bodies which need
the protection of strong homes. And the houses of some species are among
the strongest in the world.
It all starts with two termites —a king and queen.
They burrow into the earth and lay eggs. For the rest of her life, the
queen will continue to lay eggs. Gradually, an immense colony of
termites comes into being. Working together, they construct an immense
turret of hardened mud that reaches high above ground. In northern
Australia, in order to keep the termite tower cool, each of these tall
spires is made in the form of a long, upright, rectangular wedge. Each
side may be 10 feet across and 15 feet high, while only a couple feet
thick at the bottom and quite thin at the top. So the wedge points
upward. The narrow part of the termite tower lies north and south; the
broad side is toward the east and west.
The colony is quite cold by sunrise, but their home
quickly warms up because the morning light shines on its broad east
face. Then comes the hot, midday sun. But now the narrow edge of the
nest faces its burning rays. In late afternoon, as everything cools,
extra sunlight falls on the termite's home to help keep it warm through
the night.
The lesson here is that it is well, in hot areas, to
build one's house with the long side facing east and west.
But how can a blind termite, working inside the
darkness of mud cavities, know which direction to face the tower
towards? Would you know if you were as small, and weak, and blind as the
termite?
Scientists have decided that the termites use two
things to aid them in orienting their homes: (1) They use the warmth of
the sunlight. But it takes more than the sun circling overhead;
intelligent thought about how to place the slab tower in relation to
that moving orb of light is also needed. Frankly, the termite is not
smart enough to figure it out.
(2) The termite builds in relation to magnetic north.
Experiments have been carried out, in which powerful magnets were placed
around a termite nest. The termites inside were still able to face their
towers in the correct direction, but they no longer placed their nests
inside in the right places. So they use solar heat to orient the
direction of the tower, but magnetic north to tell them where, within
the darkness of the tower, to place the nests of their young.
Termite homes, located in tropical areas, have
different problems. There is too much rain and the little creatures
could be drowned out, and their homes ruined by the downpours. If you
were a blind termite, how would you solve that puzzle?
The termites do it by constructing circular towers
with conical roofs, to better shed the water as it falls. One might
consider that a simple solution. But if you were as blind as a termite,
with a brain as big as one, how would you know how to build circular
towers or conical roofs? Moreover, the eves of those conical towers
project outward, so the rain cascading off of them falls away from the
base of the tower. That takes far more thinking than a termite is able
to give to the project.
When these termites enlarge their homes, they go up
through the roof and add new sections; each section with its own new
conical roof protruding out from the side. The tower ultimately looks
like a Chinese pagoda.
The bellicose termites in Africa are warlike, hence
their name. In Nigeria, they build an underground nest containing a room
with a huge circular ceiling, large enough for a man to crawl into. It
is 10-12 feet in diameter and about 2 feet high. It is filled with
vertical shafts down to the water table. Termites go down there to
gather moist dirt to be used in enlarging their castle.
"Castle?" yes, it looks like a castle. Rising above the
termite-made underground cavern is a cluster of towers and minarets
grouped around a central spire that may rise 20 feet into the air. In
this tower is to be found floor after floor of nursery sections, fungus
gardens, food storerooms, and other areas, including the royal chambers
where the king and queen live.
The entire structure is so large that —if termites
were the size of people —their residential/office building/factory
complex would be a mile high. Could mankind devise a structure so
immense, so complicated? Yes, modern man, with his computers, written
records, architects, and engineers could make such an immense building.
But how can tiny, blind creatures —the size and intellect of worms
—manage a proportionally-sized process, much less devise it?
Before concluding this section, let us view the air
conditioning system used in this colossal structure. If you have
difficulty understanding the following description, please know that,
generation after generation, blind termites build this complicated way
—and the result is a high-quality air conditioning system:
In the center of the cavernous below-ground floor is a
massive clay pillar. This supports a thick earthen plate which forms the
ceiling of the cellar, and supports the immense weight of the central
core of the structures built in the tower.
Down in this basement cellar, the tiny-brained
termites build the cooling unit of their Central Air Conditioning System
Processor. This consists of a spiral of rings of thin vertical vanes, up
to 6 inches deep, centered around the pillar, spiraling outward and
covering the ceiling of the cavernous basement. The coils of each row of
the spiral are only an inch or so apart. The lower edge of the vanes
have holes, to increase the flow of air around and through them. The
sides of these vanes are encrusted with salt.
These delicate and complicated vanes, made of hardened
mud, absorbs moisture through the ceiling from the tower above. This
decidedly cools the incoming air, making the cellar the coldest place in
the entire building. The evaporating moisture leaves the white salts on
the vanes.
Heat, generated by the termites and their fungus
gardens in the tower, causes air from the cellar to rise through the
passageways and chambers linking the entire structure. But, as any
college-trained civil engineer would know, the cooling system is not yet
complete. A network of flues must be installed to take the hot air down
to the cooling unit in the cellar. Yes, the ignorant, blind termites
also provided those flues! From high up in the tower, a number of these
ventilation shafts run downward. As they go, they collect air from the
entire tower and send it down, past the floor plate, into the cool
cellar. As heat is produced in the various apartments of the tower, the
air flows downward through the flues, drawn by the coolness of the
cellar beneath.
The heat exchange problem has been solved, but there
is yet another one: gaseous exchange. Air may be flowing throughout the
cellar/tower, nicely cooling it, but carbon dioxide must be eliminated.
The problem here is that no casual openings to the outside are
permitted. The termites have only a few tiny entrances to the outside
world, and carefully guard each one against their many enemies. Yet they
must somehow refresh their air, Ask an engineering student to solve that
one. He has enough equations, calculators, and material specifications
that he ought to be able to provide you with a workable answer.
But those blind termites, the size of very small
worms, were applying the solution before your engineer was born, the
first college was built, and the first books were invented.
The flues are built into the outer walls of the tower.
The lining of the flues, facing the outside of the structure, are built
of specialty porous earthen material. During construction, the termites
dig small areas-or galleries-out from the flues toward the outer surface
of the outside walls. These galleries end very close to the outer
surface so gases can easily diffuse through the earth. As the stale air
travels slowly through the flues, the carbon dioxide flows out and
oxygen flows in. By the time the air has arrived at the cellar, it has
been oxygenated and refreshed. In the cellar it is cooled and then sent
back up into the tower!
Any thinking human being could, without advance
training, use the above guidelines to work out an excellent
air-conditioning system for a house. The only basic requirement is moist
heat in the upper part of the building. Engineers today call their
modified versions "passive air conditioning," but the termites
have used it ever since they came into existence.
With this system operational, the termites are able to
keep their fungus beds permanently between 30°C and 31 °C, exactly the
temperature the fungus need to grow and digest the food the termites
give them.
At this point, you might wonder why those termites
cultivate such fungus beds. While many other termites go out and eat
wood, which microbes in their stomachs digest for them, the bellicose
termites only eat fungus (they lack those stomach microbes). So they
cultivate gardens of manure in which fungus grows. The fungus grows best
within a very precise temperature range of 30-31 °C. However, the
processes of decay in the gardens produces a lot of heat (for it
operates somewhat like a compost heap). If you think about that awhile,
you will realize that this frail termite, which cannot live outside his
termite house, needs his fungus gardens, and yet, without complicated
air-conditioning, cannot maintain those beds. The termite colony needs
everything just right to begin with.
We have here another "chicken-and-the-egg"
puzzle. The world is full of them; they are all solved by the great
truth that God is the Creator. Nothing else can explain those puzzles.
You have just completed -
Chapter 8- The Creator's Handiwork- THE
EARTH
NEXT—
Go to the next file in
this series,
PREFACE FOR VOLUME 2 |