Evolution
Encyclopedia Vol. 2
Chapter 12
- THE PLANTS

We often take the plants
around us for granted, yet without them we would quickly die. They
provide man and animals with oxygen and nourishment. Only plants can
make food out of the raw materials of wafer, sunlight, and minerals.
Directly or indirectly, the rest of us eat what they produce.
There are so many wonders in plants; wonders to be
found in fruit, flowers, leaves, trunk and stems, and roots. As you
read, search for just one item that could be made by the random, harmful
effects of an occasional mutation:
CROSS POLLINATION
— Flowers Supply bees
with nectar; bees transfer pollen from one flower to another, thus
preserving the life of the plant. Bees with their long slender tongues
can reach the nectar, which other insects cannot. The tiny bee just
bristles with body hairs. As it takes the nectar, those hairs pick up
the pollen from the flower's stamens.
Some bee flowers have stamens with special levers,
triggers, or piston devices for dusting pollen on some particular spot
on the bee. Going from one flower to the next, the bee deposits that
pollen at the next flower. On each bee flight, the tiny insect somehow
knows never to gather nectar and pollen from two different species. It
always confines itself to just one species. In this way, the pollen is
always carried only to another flower of the same species.
Some flowers are pollinated by beetles. Several hold
the beetles in a trap while the stigmas receive the pollen and the
stamens sprinkle a fresh supply on the bodies of the prisoners. Then
they open an exit by which the beetle escapes.
The flowers which sunbirds pollinate, stand erect and
provide a landing platform. The petals of the flower are shaped into a
tube which exactly fits the length and curvature of the bird's bill.
Certain flowers in the tropics are pollinated by bats
which eat fruit. So the flowers give off a special fruit-like odor—but
only at night. This attracts the bats to come to them.
Some flowers are pollinated by flies. Since flies
like smelly carrion, these flowers attract them with similar odors.
When the beetle, Catonia, lights on a magnolia
flower, its weight springs a trigger like trap that releases a sudden
shower of petals that sprinkle pollen on the beetle's back. Alighting at
the next flower, its back rubs against the stigma and the pollen goes
onto it. Neither the insect nor the plant devised these things.
When the bee arrives at the Iris, it follows a
distinct marked line—a center line—on the iris flower that directs it
down to the nectar well in the center. In the process, the bee moves
under the drooping stigma which rolls pollen off its back. This stigma
is curved downward like a bent finger. Farther on in, its back picks up
a fresh supply of pollen from the anther under which it is forced to
stand in order to suck up the nectar. Meanwhile, the stigma
"finger" has straightened up—so that as the bee backs out, its
fresh pollen supply will not be scraped off and thus self-pollinate the
flower.
Certain flowers, such as the honeysuckle and petunia,
have only a faint odor during the day. In the evening, when certain
insects which should pollinate them are out, they produce a powerful
scent.
Each flower has a different story to tell about how
it attracts insects, provides a "door step" for them, presents
guide-line colors leading into the flower, and works out its various
arrangements of anther and stigma.
AJUGA PLANT— When the locusts move across North
Africa, eating everything in their path, they never touch the Ajuga
plant. This is because there is a hormone in the Ajuga which is
identical to an insect hormone in locusts and most other insects. That
particular hormone induces molting,—the shedding of the outer coat of
skin as the insect grows.
If the locust eats the Ajuga, it will cause him to
molt and shed his skin. But, because the Ajuga hormone is five times
stronger than that found in locusts, it would pop his skin too fast. So
locusts that eat the Ajuga quickly lose the skin around their mouths and
they starve to death. Most leave it alone.
FIG AND WASP—When California planters introduced
the Turkish fig, they found it bore no fruit. The trees were covered
with flower buds which dropped off without ripening. The problem was
that they had brought no fig wasps for that particular fig tree. American entomologists went to
Smyrna in Turkey and brought back the fig wasp for that particular fig
tree. They then named the fine-tasting fruit the Calimyrna.
Most experts believe that every type of fig in the
world has its own particular wasp. But others say that some fig species
do not need wasps (for example, Black mission and Adriatic figs). At any
rate, those that do could not survive without their particular wasp.
This wasp spends its life pollinating that one type of fig tree. The
tree in turn provides a home for the young of the fig wasp.
Here, briefly, is how the female wasp does her work;
the story of the male fig wasp is equally complex.
There are two kinds of figs: (1) the male fig (caprifig)
which is very small and not for eating. It grows the pollen which
produces the other kind of fig. (2) the female fig (the Calimyrna,
Smyrna, or Turkish fig),
which is so delicious. Because the flower parts of the fig are all
inside the fig, there is no possibility that wind could pollinate the
flowers. The little wasp must do it, or there will be no figs and when
the fig tree dies, it will leave no descendents.
The fig wasp is the size of a fruit
fly—about
1 /16 inch [.159 cm] long. It crawls into the fig through a hole in the
male fig, and there it lays its eggs. The fig wasps hatch from the eggs,
and the young feed upon pollen inside the fig, which itself is odd since
most flowers have their pollen on the outside of the flower, not inside
the fruit. Then, after mating, the tiny wasps leave their birthplace.
They are covered with pollen as they emerge from the fig. The little
female wasp must work quickly for it only lives 12 hours. Going from fig
to fig, it enters through the small hole at the end, pushing its way
through a row of staminate flowers, and comes to rest on a bed of
pistillate flowers found in the center of the fig. It is searching for
the male fig, so it can lay its eggs, but it enters every fig, thus
pollinating the female figs in the process. That is an important fact:
the little wasp knows that it must enter every fig it finds, for it
cannot tell them apart from the outside; yet once inside, it knows it
can only lay its eggs inside the male fig.
The female wasp deposits eggs which hatch into larvae
and which, in due time become a new generation of wasps.
These mate with each other inside the fig. Leaving
it, they are dusted with pollen from the staminate flowers surrounding
the entrance. The fig wasps fly away and search for another fig tree of
the same type to repeat the process. They spend their brief lives going
from one fig tree to another, pollinating each one,—but they never go to
a fig tree that is not of the same type. If they did, we would only have
one type of fig, but as it is we have several types. No one
knows how they manage to find their particular fig tree, and the
tiny hole of the fig on that tree.
Wind cannot pollinate figs, for the pollen cannot get
inside the tiny hole. But without pollination, there would be no fruit, no seeds, no fig
trees. From the very beginning, there had to be both fig wasps and the
fig tree with its fruit. Otherwise we would have no figs and fig wasps
today.
YUCCA AND MOTH
—Without
a tiny white moth —the pronuba moth,—the large yucca would die. This
desert plant appears like a cluster of sharp swords pointing out in all
directions. Out of its center arises the stalk of a bright, beautiful
flower that looks something like a white lily.
Hiding in the ground is a small moth which never
comes out during the day. It only comes out at night—on a certain night.
The flower, in turn, only blooms at certain times of
the year—and only at night. When it blooms, immediately the pronuba
moths break out of their cocoons beneath the sand.
What brought them out
of their hiding places down in the desert sand at exactly that moment?
How could a tiny wasp in the ground know that a flower had bloomed? No
one knows. Struggling up out of the sand, the tiny female moth flies up
into the air and circles around until it catches the scent of the
flower, and then goes to it.
Arriving there, the moth, which has eaten
nothing for a long time, ignores the nectar but instead goes to the top
of the stamens of the first flower and, with its tiny feet, carefully
scrapes together a wad of pollen that is three times as big as its head.
Holding onto it with jaws and legs (it was born with specially enlarged
ones for this purpose),—the insect flies to another yucca plant. Backing
down into the heart of a flower, the moth pierces a hole with an
egg-laying needle (a lancelike ovipositor) and lays eggs among the seed
cells in the green pod at the base of the pistil.
Next, the insect climbs to the top of the pistil on
that same flower. It has a cavity just the right size to receive the wad
of pollen. Carefully the moth stuffs the cavity with the pollen. The top
of the pistil looks like there is a funnel-shaped opening within it.
Into that opening the moth pushes the pollen. By doing this, seeds will
grow at the base of that particular pistil. But it was at that same base
that the moth laid its eggs. Some of those seeds will provide food for
the baby insects when they are later born. If the moth pushed the pollen
into the top of the wrong pistil, its babies would die.
Time passes as the pronuba eggs mature and the yucca
seeds ripen. When the moth's larvae (caterpillers) emerge from their
eggs, they are surrounded by delicious food. They eat and grow larger.
But they never eat all the seeds. Their nutritional needs never require
eating all the seeds at the base of that particular pistil.
Then, about two months after hatching, each one cuts
a hole through the pod, spins a silk thread, and lets itself down to the
ground. Arriving there, it digs a hole, crawls in,—and waits about ten
months till the next flowering.
But what happened to that mother moth? After flying
to one flower, taking pollen to another, laying eggs and pollinating the
pistil,—the little moth
dies. After leaving the ground it never once eats, but only does its
work of providing for the future of its babies and the yucca plant.
There is still more: Each species of Yucca plant has
its own special moth! The flower is so constructed that it can only be
pollinated by one particular type of moth.
During certain years, the flowers do not appear on
the plants. If the moths came out at that time, they would die—and the
Yuccas would die later on. But, instead, the moths only come out when
the flowers appear—even if the moths have to wait till the second or
third year to come out of the ground!
"Could blind chance have achieved such
perfection [referring to the Yucca plant and the
pronubia moth]?."— 'Ernsi Mayr, "Accident or Design: the
Paradox of Evolution, " in The Evolution of Living Things (1962),
p. 3.
"It is a considerable strain on one's
credulity to assume that the famous yucca moth case could
result from random mutations."— 'Ernst Mayr, Systematics and the
Origin of Species (1942), P. 296.
THORNY ACACIA— The thorny acacia tree of central
Africa can tell when animals are feeding too heavily on it. When that
happens, it begins producing a chemical called tannin
k. The tannin combines with
other chemicals in the leaves, producing a bad taste. Scientists found
that the tannin level is normally quite low, but within 15 minutes after
leaf damage, tannin levels in the leaves nearly doubled. In addition,
they discovered that when this happens, the tree gives off an odor,
warning other nearby acacia trees to be on guard. In response, they
immediately begin producing more tannin in their leaves also!
LADYSLIPPER— The Lady's Slipper Orchid
has two
stamens. The lip is shaped like a smooth slipper with in-rolled edges, so
the insect cannot get out by the way it entered. So it must move toward
the back, or point of attachment to the stem, where there are two small
exits. Heading that way, the creature must first pass beneath a stigma
which takes pollen from the insect. Then it must brush past one or the
other of the two stamens which sprinkle more on it. Leaving the flower,
the insect never goes to another flower on the same plant, because only
one flower will be open at any given time. In this way self-pollination
does not occur.
RICE —Rice is a land plant and must have oxygen in
its root to survive. Yet it must be submerged in water—often 15 feet [46
dm] deep—in order to grow and seed. The rice must grow and keep above
the water! In flood-prone areas, rice grows as much as a foot a day in
order to keep its topmost leaves above the surface of the flooded rice
paddy. The rice plant draws in water through its exposed leaves, as well
as through a sheath of air surrounding its submerged stalk.
Rice gives off one carbon dioxide molecule for every
oxygen molecule it takes in. But, because the carbon dioxide dissolves
more quickly in water than does oxygen, a vacuum is created within the
plant which pulls in yet more air! You could not draw air through a hose
to a depth of 15 feet (46 dm], but the rice plant can draw air down its
stalk that far, because of that partial vacuum.
PLANT ODDITIES— The yellow evening primrose opens
only at dusk,—and so swiftly that it can be seen and heard. The buds
sound like popping soap bubbles as they burst.
Seeds of the African baobab tree sprout more easily
if they are first eaten by a baboon and passed through his digestive
tract. Its digestive juices erode the tough seed coat, permitting water
to penetrate more readily.
In a single growing season, 10 small water hyacinths
can increase to more than 600,000 plants, and form a mat of thick
vegetation an acre in size and weighing 180 tons [163 mt]!
The stems of the blue-eyed grass, a type of wild
iris, are not strong enough to support more than one blossom at a time.
So one flower blooms each morning, and then dies that night so that
another can bloom the next day.
Bamboo can grow three feet [9 dm] in 24 hours.
The ocean contains eighty-five percent of all the
plant life in the world.
A typical plant or tree receives about 10 percent of
its nutrition from the ground; the rest comes from the atmosphere and
sunlight.
The giant water lily, victoria regia, has leaves so
large that a small child could sit on it without its sinking. The leaves
are eight feet across.
Lichens have been found on bare rocks in Antarctica
as close as 264 miles [424.85 km] to the South Pole. No other
plant or animal life lives that near to the South Pole.
The dwarf mistletoe in America builds up hydraulic
pressure within it—equal to that found in a truck tire! It does this in
order to use that water pressure to catapult its seeds out to a distance
of almost 50 feet [152 dm] at a speed of close to 60 miles (96.5 km] per
hour. The dwarf mistletoe is a water cannon!
Tiny discs of chlorophyll move about within plant
cells and adjust for different light and heat conditions. When the
sunlight is too strong, the little discs turn edgewise! On an overcast
day, they lie as parallel to the sky as they can in order to take in the
most light.
Some plants die as soon as they have flowered, while
some trees live up to 4,000 years. There is a bamboo plant in the
mountains of Jamaica which takes 32 years to mature, and then flowers
once and dies.
Puffball and mushroom spores have been found in large
amounts 35,000 feet [10,668 m] in the air.
The Mediterranean squirting cucumber uses water
pressure to shoot its seeds 40 feet (122 dm]
away.
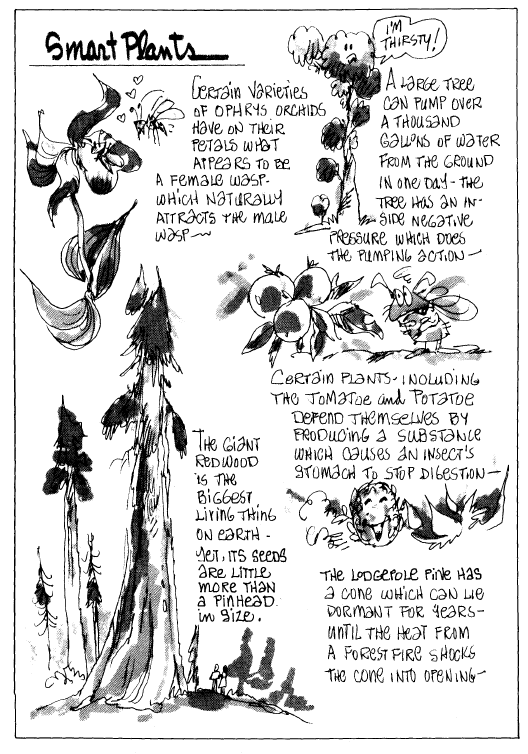
GALL—When the gallfly lays its eggs in the leaf or
stem of a plant, a large ball-like growth occurs. This gall, as the
growth is called, serves as a home for—and gives food to—the developing
insect. Galls are of great variety, both in shape and color. Several
different types of insects produce galls in plants as "nests"
for their young.
Until recent decades it was thought that the gall was
produced by the plant as a means of protecting itself from injury. But
it is now known that the gall was produced because the insect injected a
plant growth hormone into the leaf or stem! This is incredible; an insect
manufacturing plant hormones in its body! Once injected, the
hormone causes the growth to occur. How could an insect invent plant
hormones?
THE COLOR OF PLANTS—Light from the sun contains all the colors of the rainbow.
When it strikes the plant, the plant absorbs the red and purple rays and
uses them in photosynthesis. "Photosynthesis" is that
marvelous action by which a chunk of sunlight and a chunk of water are
transformed into carbohydrates (simple and complex sugars).
Because the plant absorbed the red and purple rays,
the yellow and green ones are reflected back outward. This gives the
landscape its great beauty.
It could have been the other way around, and the
plant could have absorbed the yellow and green, and reflected the red
and purple! Instead of the restful colors, we could have been surrounded
with violent ones. If red, yellow, and green had been absorbed, we would
see deep blue and violet in the plants. This would have been too
depressing. If green, blue, and violet had been absorbed, we would only
see brilliant reds and oranges all about us. This would have been too
exciting and overstimulating to the nerves.
Instead we have soothing green as the predominate
color of vegetation.
MADRONE AND MANZANITA —In California, the madrones
and manzanitas have thick, heavy leaves that can endure the cold
winters. In wintertime they are broad-leaved evergreens. But in
mid-summer, when their leaves are in the greatest danger of drought,
they shed their leaves. When the stem leaf breaks off and the leaf
falls, at the base of the petiole is to be found a corky layer. This
seals off the plant, so that, even though the leaf is gone, no fluids
will evaporate out through that opening. This serves as a plug to stop
the passage of water from the stem. Otherwise these large bushes would
dry out and die.
AIR PLANTS— Some plants grow on trees and not in
the soil. But they are not parasites, for their nourishment is not
obtained from the tree, but from the air! Air plants, such as tropical
orchids, have a mass of tissue around the roots which absorbs water from
the air.
EELGRASS—
Eelgrass grows submerged in the shallow
water of bays and estuaries near the seacoast. It is like regular grass,
but much longer. Eelgrass is the only flowering plant that blooms
underwater! Its pollen is shed into the water, and is carried by
currents to other nearby plants.
POLLINATION—Night-blooming flowers are white or
yellow, so they will be visible in the darkness. To make sure they will
be found by pollinating insects, they are also provided with fragrant
odors. Many of them are closed and odorless during the daytime.
Wind-pollinated flowers do not have brightly colored
corollas. They do not need them, since they do not need to attract
insects to pollinate them.
The brilliant flowers are generally pollinated by
insects. These flowers are also equipped with odors emitted into the air
during the day in order to attract insect visitors.
Sometimes the petals have guidelines (stripes) to
help insects land and enter the flower at just the right place. In order
to get into the nectar pots, the insect first passes by the anthers and
stigma, which are always in front of the nectar pots.
AUSTRALIAN PLANT ROOTS —In
the Australian back
country (the "bush") the natives search for a certain small
plant which, although it only has about 4 inches [10.16 cm] of leaves
above the soil, has roots which are larger than footballs and full of
water. These roots are reservoirs to be drawn upon during the almost
continuous dry weather in those regions. Finding these, the aborigines
split them open and drink the water.
There is another desert plant in the Australian
"out-back" which has roots which are shaped like long strings
of sausages, 10 to 18 feet [30-55 dm] in length. Finding them, the
natives will hang them on trees so that the water will run out.
MALLOW—As do many other plants, the leaves of the
mallow weed follow the movement of the sun across the sky. Then, as soon
as the sun sets, all the mallow plants turn and face east to where it
will rise in the morning.
DUVANA—The Duvana dependens grows a
special gall, an enlargement, on its stem which is of no use to the
plant itself. But the moth Cecidosis eremita needs that gall in
order to survive. It comes to the Duvana and lays its eggs in that gall.
KELP—The California Kelp is to be found a halfmile
off the coast of California. It grows in giant kelp forests which are 25
feet [76 dm] tall. Millions of baby fish and crabs grow up in the
tangled leaves of these forests. Thousands of other fish and sea
creatures live there also. It provides food, shelter, and nesting places
for millions.
In addition, bald eagles swoop down and obtain their
food from creatures at the top of this forest, and harbor seals get
their food from it also. But most of the creatures in the kelp forest
are not caught and they grow up and replenish the marine ecological system.
All the creatures eating the kelp leaves are no
problem; it simply grows more leaves. Yet the spiny sea urchin is
different; it is a menace, for it cuts through the kelp stem. But the
sea otter is in the kelp forest also, and it thoroughly enjoys eating
sea urchins.
Thus the balance continues. Only man can upset it by
over harvesting the kelp or killing the sea otters.
COAST REDWOOD—This tree on the Northern
California coast sprouts from one of the smallest of seeds, yet grows
taller than a 35-story building or the Statue of Liberty. It easily
reaches 350 feet [107 m] in height, and the tallest one is 375 feet [114
m]. Twenty feet across and 65 feet around, its roots only go down 3 or 4
feet [91-122 cm] , but they spread out 80 feet [244 dm] on each side.
The first branch is over 150 feet [457 dm] up, and its bark is over a
foot thick. It more than a thousand years old (while the giant Sequoia
in the Sierra Nevada Mountains is 4,000 years old).
These 350-foot [114 m] high giants along the Northern
California coast rain down their tiny seeds, but most of them are eaten.
Only 10 percent of the redwood in the forests came from seeds; 90
percent came from sprouts.
At the base of each tree, and surrounding it in a
circular collar, are wartlike growths from its roots. These are called
"redwood burls." If a tree gets into serious trouble from
fire, bugs, etc. , it will send a hormone message to the burls and
immediately they will sprout! As many as 100 will sprout up around the
parent tree. In 20 years, each sprout will be 50 feet [152 dm] tall and
8 feet [24 dm] in diameter.
The coast redwood grows in only one place in the
world: the northern California coast. This is partly due to the moderate
climate, but another reason is the fog that comes in nearly every day
during the hot and dry part of the year and drips down,
moistening trees, ground, and roots. Without that fog the coast redwood
could not live.
SORREL—On the ground beneath the tall coast
redwood is the tiny sorrel. This is a three-leaved plant which is
designed to grow in the continuous shadow of the giant trees, above
which the sky is frequently overcast with fog. The small leaves of the
sorrel lie flat catching every bit of skylight they can.
But occasionally the sunlight shines through a patch
in the tree tops—and hits those leaves. Immediately, the little plant
must do something or it will die. This is because it is designed for
shadowed living, not sunlit living! Within a short time, the sunlight
will wither the plant and it will perish.
Quickly, the little plant folds its three leaves
upright—like shutting an umbrella. This shuts out the sunlight and heat.
Only exposed now is the bottom side, which on most plants also has
chlorophyll, but on the sorrel has a purple screen to protect the plant.
As an added protection, sorrel primarily grows by
sending out runners. In this way, many plants are connected underground.
They all cooperate with one another in an emergency and if one gets into
a patch of sunlight, it sends out a message to the others and they send
more moisture to it.
ANCHOMANES— Plants of the Anchomanes genus only
have one leaf, produce heat like warmblooded animals, and make insect
food. Anchomanes only grow in Africa. Above the root, there is only one
leaf,—but it is about 20 feet [61 dm] tall and 6 feet [183 cm] wide!
When it blooms, it generates heat by burning carbohydrates. The flower
only opens at night, has no scent, and is a dark maroon color. Yet
somehow it is located by a certain pollinating beetle. Arriving at the
flower, the beetles feed on small granular lumps on the underside of the
flower, made especially for them. Soon large numbers of beetles have
arrived, and they mate and lay their eggs on the flower, where the young
develop without damaging the plant.
DUTCHMAN'S PIPE—The Dutchman's
Pipe has a tubular
leaf that wraps around its flower. This leaf is coated with wax. Certain
insects are attracted by the strong odor of the flower and land on the
leaf. As soon as an insect does, it slides down its slippery sides into
a chamber at the bottom. There, the ripe stigmas receive the pollen that
the insect brought with it, and pollination takes place.
Three days then pass by with the insect trapped by
hairs near the bottom and the wax farther up the sides. After that, the
flower's own pollen ripens—and dusts the insect. As soon as that
happens, the imprisoning hairs wilt and the waxed slide of the funnel—like flower bends over until it is nearly level. The insect now
walks out with his supply of pollen—and flies off to do it again. One
might think that the insect could starve living like that, but all the
while it is inside the flower it is feeding on a feast of stored nectar.
CORNSILK TUBES—Cornsllk is that golden hair which
protrudes out of an ear of corn. When a single bit of corn pollen lands
on the pink silk at the top of the ear, it stays there because the silk
thread is sticky. That extremely tiny pollen grain then begins making a
tube that eats its way into the thread. If the grain lands near the
outer end of the silk, this tube may lengthen by ten inches as it
travels down the inside of the thread of "silk." It is
striving to reach an egg cell far below at the base of the thread.
Arriving there, it will slowly transform it into a kernel of corn.
LONG-LIVED POLLEN—Each grain of plant pollen is
enclosed in a case that is almost indestructible. It does not decay as
do the other parts of the plant. Pollen grains thrown out by plants have
survived for long periods of time. Even after they finally die, the
outer hull continues to retain its same shape. This is why pollen can be
found wherever man searches for fossils. Pollen grains have
been found in the lowest strata—the Cambrian —of Grand Canyon, showing
that plants were living and thriving way back in the beginning. And
would we expect otherwise? Without plants in the beginning, none of us
would be alive today!
VARIGATED POLLEN—There are over half a million
flowering plants in the world, plus large numbers of trees, bushes and
other plants. Yet every species of plant in the world that produces
pollen—makes a uniquely shaped pollen grain! No two plant types form the
shape of their pollen in exactly the same way.
Under a microscope, a grain of pollen looks like an
exquisite jewel. The grains may look like disks, footballs, canoes,
dumbbells, crystals, etc., but no two will be exactly alike unless they
come from the same species.
OPHRYS ORCHIDS—Certain varieties of the Ophrys
orchid have on their petals what appears to be a three-dimensional
picture of a female wasp, complete with eyes, antennae and wings. The
petal even gives off the odor of a female in mating condition! When the
male arrives to mate, he only pollinates the flower.
MILKWEED—The milkweed produces glycosides which
provide no nourishment to the plant, but instead protect the monarch
butterfly which feeds upon the plant. Without that protection, the
Monarch could not survive, for it only eats from the milkweed during its
lifetime.
Certain plants, including the milkweed, produce a
sticky fluid which protect them against aphids. When aphids come to
dine, they suck out some of this fluid—and it causes a sticky,
coagulated mess in their tubes and stomach. The aphids either depart
immediately or die.
RABBIT AND AMANITA— The Amanita
is one of the
deadliest things in the world. Mushroom experts declare that the Amanita
is the only mushroom in the world which will kill a person.
Unfortunately, it comes in many different colors. (In America it is most
commonly seen in the "death angel," a pure white variety; if
you see any growing on your lawn or in the woods,—warn your children to
leave them alone!) If no antidote is given within 30 minutes, death will
follow.
But the rabbit can eat the Amanita without
experiencing any ill effects. The poison in this mushroom (phallin)
causes it no harm. No one knows
why the rabbit is unaffected by one of most powerful poisons known to
mankind.
MOVING THE POLLEN AROUND—
In
most instances, a
plant places the fresh new supply of pollen on exactly the place on the
insect where, but a moment before, it removed the pollen from the
previous plant. Yet there are exceptions. Sometimes the pollen is
collected by the plant in a different location on the insect's body than
it was deposited by the previous flower. So what does the insect do? In
such cases a certain type of insect will pollinate that particular species of
flower—and before entering the second flower, it will obligingly shift
its load to the proper flower pick-up point!
SCORPIURUS—The pod of the Scorpiurus, resembles a
nice, tasty centipede. Sighting it, birds grab it and fly off with it.
Gripping it in their teeth, the pod breaks open and scatters seeds as
they go. Landing, they find that it is not edible and spit out the
now-empty pod.
BUCKET ORCHID—This orchid has a slightly
fermented nectar which makes the bee wobbly on its feet, causing it to
slip into a bucket of liquid. The only exit is along a route that causes
it to wriggle under a rod that dusts the bee with pollen.
ANT AND ACACIA TREE—Acacia
trees have beautiful
flowers in the spring. If you were to look closely you would find the
Pseudomyrma ant in them. It lives in the Acacia tree and feeds on
special fruit on the leaves. This fruit does not contain any seeds, and
has nothing to do with producing more Acacia trees. It is only there to
provide food for the Pseudomyrma ant.
But, because that fruit is there,
the little ant is there. In turn, the ant travels about over the tree
and chases off—or eats—other insects which would eat the Acacia leaves.
They even destroy climbing vines which would kill the tree, as well as
small nearby trees which could grow and shade their special tree. Each
tree has its own resident colony of ants which feed on and protect it.
This acacia is the only plant in the world that produces the animal
starch, glycogen.
One type of acacia has swollen thorns in which the
ants hollow out nests. When an ant queen discovers an unoccupied tree,
she burrows into a green thorn and lays her eggs. The larvae are fed
with carbohydrates from the leaf tips. Eventually the colony of ants on
one tree may number 30,000, when all the thorns are occupied. Then the
colony splits into two, and part of it swarms to another tree.
CAMBIUM LAYER—A marvelous outer circle of cells
is on every tree. It is called the cambium. This is the growing edge of
the tree. On the inside, it makes xylem
tubes—thus increasing the amount
of wood in the tree. On the outside of the cambium layer, it makes phloem
tubes—which adds on more outer
stem or bark. No scientist can explain where the cambium came from. But
without it, there would be no plants
LEAVES—What are leaves? Each one is a power station.
The leaf includes chloroplasts, guard cells, special chemicals, and
much, much more. Filled with tubing through which fluids flow, it has
five layers of water-proof coatings, and the top coat is akin to
varnish.
The location of each leaf dovetails into the others,
so that each leaf can obtain as much sunlight as possible. In order to
do that, each leaf must be moved into the best position relative to the
others. Where are the brains to do this? in the leaf? In the branch?
Each leaf is a sunlight machine. It takes in sunlight—and, together with minerals and water from the roots, the plant
turns out all the basic food used by every plant and animal in the
entire world! Without the leaf, we would all quickly perish.
Man makes solar panals to catch the sunlight. These
are spread out so each plate will receive sunlight. Imagine how much
space the leaves of a tree would need, if they were spread out flat all
over the ground? God's way is much more efficient. All the life on our
planet is fueled by solar power!
CHLOROPLASTS—Scientists
estimate that over 400 million-million horsepower of solar energy
reaches the earth every day. Photosynthesis is the process by which
sunlight is transformed into carbohydrates. This takes place in the
chloroplasts. Each one is lens-shaped, something like an almost flat
cone with the rounded part on the upper side. Sunlight enters from
above.
Inside the chloroplast are tiny cylinders that look
something like the small circular batteries used in hearing aids and
small electrical devices. These are called lamellae, and is actually a
stack of several disk-shaped thylakoids.
Each thylakoid is the shape of a
coin. Several of these are stacked on top of each other, and this makes
a single lamelium. A small narrow band connects each stack to another
stack. They look like they are all wired like a bunch of batteries.
Sunlight is processed by chlorophyll
in those stacks, and then stored
there as chemical energy in the form of sugar molecules. Chlorophyll,
itself, is very complicated and never exists outside of the plant, just
as DNA and ten thousands of other chemical structures never exist
outside plants and/or animals. If they are not found outside, how did
they ever get inside?
BLUE-GREEN ALGAE— This IS
probably the simplest of the
plants. Yet its structure, functions, and chemistry is awesome,—and all
of it had to be present and functioning from the very beginning.
Blue-green algae produce more oxygen than almost
anything else in the world. They are found in oceans, waterways, and
lakes. They photosynthesize and respire almost like higher plants. Some
of them can fix nitrogen from the air, so that their food requirements
are minimal. These algae serve, under the name plankton,
as the basic food for animal
life in fresh and salt water throughout the entire world.
MITOCHONDRION AND
ATP—Mitochondria
within the plant cell are little
capsule-shaped containers. They take in sugars, fats and even proteins,
which are made elsewhere in the plant, and change these substances into
ATP.
Each molecule of ATP is a miniature storage battery
and contains electrical power. ATP molecules are stored in the plant and
used whenever needed for a variety of
purposes—whenever energy is
needed. ATP is an amazing substance.
PLANT BLOOD— A drop of blood contains about a
hundred million red cells. Each of these small doughnut-shaped discs is
covered with one of the largest and most complex molecules in nature: hemoglobin.
Hemoglobin has been called a
"molecular lung," for it is an oxygen processer just as is the
lung. Remove the iron from the center of hemoglobin and place magnesium
in its place,—and you have chlorophyll, which is so important to the
life of the plant.
Are people related to peas? A nitrogen-fixing
bacterium, Rhizobium, in
the root nodules of peas, enables the legumes to make hemoglobin genes.
Rhizobium has hemoglobin genes also. What is hemoglobin doing in peas
and bacteria!
But that need not surprise you. The water flea,
Daphnia,
has hemoglobin also! Then
consider the ice-fish, which lives in antarctic waters averaging 2°C
[35.6°F). It has no hemoglobin—but instead has a form of antifreeze
which circulates through its veins!
RYE PLANTS—A single plant of winter rye has roots
one hundred times greater than all the parts growing above ground. Its
regular brown roots grow three miles of new roots per day. In addition,
billions of microscopic white root hairs branch out from them, sliding
through spaces between grains of soil. Adding these to the already large
total, scientists decided that rye adds 53 miles [85 km) of additional
roots per day.
SEEDS UNLIMITED—Plants pour out seeds. A single
plant of red clover only a few inches tall turns out 500 copies of
itself. The weedy crabgrass makes 90,000 seeds on each plant. Pigweed
produces a million seeds per plant.
One orchid was estimated to grow 3,770,000 seeds on a
single plant. Orchids grow high up in jungle trees, and their seed must
find a limb which is wet and the bark slightly decayed. So millions of
seeds, as fine as the finest powder float off into the air.
Dandelion seeds come equipped with parachutes. Maple
seeds have wings and flutter off like butterflies. Some water plants
produce seeds with air-filled floats. When released, they just sail
away, as the wind blows them along.
Other plants have pods that snap open and shoot their
seeds out as from guns. Witch hazel pods gradually press tighter and
tighter against their slippery seeds—until out they pop and travel some
distance before landing. As the squirting cucumber grows, its pod
thickens inwardly. The fluid center comes under ever-increased pressure
till—bang! and the pressure becomes so great that the seeds shoot out
like a cork from a bottle.
A small number of dry bean seeds,
accidentally left
under a concrete sidewalk, will, when they get wet, swell with such
power that they will break the concrete.
Some desert seeds just lie on the ground and
refuse to sprout—till a desert rain of a half inch or
more occurs. Only a heavy rain will provide them with enough moisture to
go through their brief cycle of life. Then they go to work fast!
Scientists tried to figure out a problem here: Why is
it these seeds will not sprout if they are only wet from below? Why must
they also be soaked from above! The reason is this: The desert soil has
too many salts in it—salts that will prevent the seeds from sprouting.
So a rain is needed to wash down the salts so that the seeds can sprout
and grow.
KNOBCONE PINE SEEDS— The knobcone pine has fire
insurance. Unlike most pine trees, which open their cones and let the
seeds slide out when ripe, the knobcone holds its ripe seeds sealed
inside the cone. This cone is almost as hard as rock and will remain on
the tree for as long as 50 years. These cones hug the trunk of the tree,
so they are eventually swallowed by bark growing around them. But inside
those cones, the seeds are still alive. Even if the tree dies, the
waiting seeds continue to be alive. Still more time passes, and then a
forest fire occurs.
Since only a fire can release those seeds, they now
spring into action! As the fire passes over the tree, the cones explode
like popcorn. This explosion flings seeds everywhere, and they take root
in the ashes after they have been cooled and wet by rain. In this way,
these young trees grow and protect the forest floor from erosion. Later,
other trees reforest the area along with them.
TRAVELING SEEDS— Some seeds are inside fruit, and
when eaten the seeds reach the ground and sprout. Acorns are carried off
by squirrels who know enough to bury them, and then forget where many of
them are so they can sprout.
The burdock seed has big hooks that hitchhike on
passing animals and people. Seeds of burr, marigold, ticktrefoil, or
Spanish needles, travel in the same way.
Other seeds rely not on hooks but sticky surfaces.
Still others are coated with oil, so ants carry them off to their
underground homes where some of them will sprout.
Then there are the seeds which are part of such
contraptions as slingshots, catapults, spring mechanisms, exploding
parts, and cannons.
What about the overcoat seed on the wild oat? It has
an overcoat called an awn
which looks like a partly-bent
leg of a grasshopper. On warm, dry days, the leg suddenly straightens
with such force that the seed is lifted over rough ground and partially
burrows itself into the ground.
SMALLEST TO
THE LARGEST— One of the
very smallest of the seeds, eventually grows into the biggest living
thing on earth (and probably the heaviest too). The giant sequoia of the
Sierra Nevada range grows over 300 feet [91 m] high, with a diameter
which may be 36 feet [11 m]. One tree may contain enough wood to build
50 six-room houses. The bark is two feet [61 cm] thick, and its roots cover 3 or 4 acres [1.2-1.6 ha].
Yet its seeds are little more than a pinhead
surrounded by tiny wings.
ROOTS—Green leaves feed the world, but they cannot
function without the roots. Each tiny rootlet has a small cap protecting
its end as it grows outward. Each tiny cap is lubricated with oil.
Continually these rootlets, covered by caps, are pushing through the
soil.
Behind them, root hairs absorb water and minerals,
which travel up extremely small channels in the sapwood. This fluid
moves upward at 200 feet [610 dm] per hour! Up and up it goes, till it
reaches the factories in the leaves. Here sugars and amino acids are
made, which are then sent throughout the tree to nourish it.
Large amounts of excess water evaporates from the
leaves into the atmosphere, which rise upward and form clouds to later
fall as rain and help plants, animals, and man.
ULTRA-VIOLET PLANTS—Certain
flowers, such as Jasminium
primulinum, have been found to
have hidden patterns, generally on the rear of the flowers, which can be
seen only under ultraviolet light. After careful investigation,
scientists have decided that certain insects find these flowers by
ultra-violet light!
It is known that some insects (how many has not yet
been determined) can see ultra-violet light, at least the near
ultra-violet spectrum. For example, bees can see UV light. No one has so
far been able to figure out how they do it.
TREE PUMP—On a warm summer day, a large tree may
pump over a thousand gallons [3785 I] of water from the ground, up
through its trunk and branches, and out into its leaves. That is four
tons [3.6 mt] of water in one day! Drop by drop, the water is drawn out
of the soil by the roots. But it is what is happening in the top of the
tree—30 to 100 feet [91-305 dm] up in the air—that causes the water to
be taken on up. As water evaporates from the leaves, it produces a
negative pressure inside the tree's tubing If you were to cut one of
those vessels, a hissing sound—of air rushing in—could be heard.
Negative pressures as low as negative 20 atmospheres have been found
high in trees. This is what draws the water up the tree.
REPELLING AN
INSECT THROUGH ITS STOMACH—Certain plants, including
the tomato and potato, have special ways of defending themselves against
insects. If a leaf is damaged as an insect begins to eat it, the plant
produces a considerable concentration of a substance which causes
problems in the insect's stomach so it cannot digest its food. The
substance causes the insect's stomach digestive juices—proteinases—to
stop flowing! Henceforth, the insect leaves that plant alone.
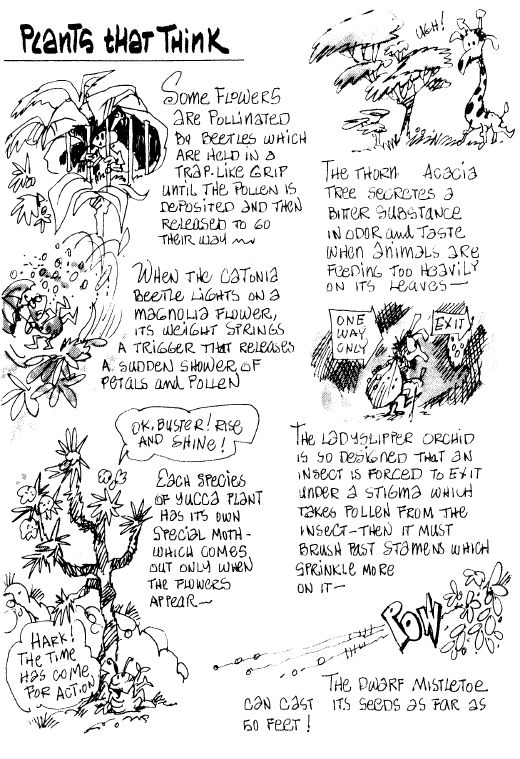
TITYRA AND
CASEARIA —In the forests Of Costa
Rica, there is a bird and a tree that work together for mutual benefit.
Most birds eat fruit wherever they might find it, dropping the seeds at
the base of the tree where most of them die. But the tityra bird
consistently depends on the Casearia corymbosa tree for food. In turn,
that tree depends on the tityira to scatter its seeds so more Casearia
trees will grow.
Two species of tityra birds pluck Casearia
fruit—but
immediately fly off with the fruit some distance from the parent tree,
dropping the seed where it has a much better chance of successful
germination and growth.
H.F. Howe, the plant researcher who discovered this
relationship, commmented that it is clear that without either the bird
or the tree, the other would perish.
FIRE SEED—Many trees depend on forest fires to
propogate them. They lay there for years until a fire passes through,
and then, afterward, they sprout. The lodgepole pine, on the West Coast,
has special fire insurance. It produces two types of cones. The first
cone opens and releases its seeds at the regular time in the spring. But
the second remains unopened, falls to the ground and lies dormant for
years. When a forest fire occurs, it shocks those sealed cones into
opening. The seeds fall out and a new forest begins growing.
BULL'S HORN ACACIA AND THE
ANTS—The myrmecophytes are plants in South America which are
inhabited by ants. A species of ferocious stinging ants come to these
trees and make their home there. They pierce the thorns to use as nests,
and eat small green bumps on the twigs and little brown nubbins on the
leaf tips. Thus these ants get both food and shelter from these trees.
In return, they protect that tree from encrouching
insects, goats, and other foraging creatures of various sizes. In
addition, the ants make regular forays in all directions from their tree—and destroy strangler vines which would kill it, and nip off every
green shoot that might threaten to encroach upon the space reserved for
their tree to grow and thrive.
To see what would happen to the tree if it lost its
ants, scientists carefully killed all the ants on several of these
trees, and then made sure that no more ants arrived. Within 2 to 15
months the trees died,—either eaten by foraging animals and insects or
suffocated by the vegetation of the surrounding jungle.
MANGROVE'S SALT-FREE DIET—The mangrove tree is
one of the few trees that grows in salt water. Its roots suck up the
seawater, yet the salt in that water would kill the tree within hours if
taken up through the roots and sent up the trunk into the leaves. To solve this problem, the roots carefully filter
out
the salt by passing it through special membranes
that remove it.
One species of mangrove does it differently:
Partly-filtered sea water is sent up to the leaves, where it passes
through small glands on the underside of leaves, where excess salt is taken out
and dropped through tiny holes in the bottom of the leaf.
CAP-THROWING FUNGUS—The cap-throwing fungus has a
built-in clock mechanism that is keyed to the movements of the sun.
Throughout the day it turns with the sun. Then, the next morning at
about 9 a.m., it knows that the best time has come to throw out its
spores. In response to its light-sensing system, the cap-throwing fungus
explodes its top—and hurls out its spores. Upon landing, they are picked
up by passing animals and carried elsewhere. A glue coating on the
spores aids in this process.
PLANT BLADDERWORT—The common bladderwort (Utricularia
vulgarls) lives in ponds. It is shaped like a funnel and spends its time
snaring small aquatic insects and crustacea. Its mouth has a hinged
trapdoor with a very sensitive trigger. To set the trap, the sac of the
funnel is collapsed by pushing all the water out of it.
Along the outer edge of the funnel top are trigger
hairs and also a hinged trapdoor. When a swimming insect or plankton
touches the hair trigger, the bladder—the inside of the funnel or body
of the bladderwort—expands in 1/50th of a second! This produces a strong
vacuum which sucks the insect into the funnel. The vacuum pressure
thrusts the entire bladderwort forward a distance.
THE FIBONACCI SERIES—Plants
and many other things
in nature are keyed to various involved mathematical formulas, one of
which is the Fibonacci series.
Leonardo of Pisa, nicknamed Fibonacci (c. 1170-1230 A. D.) discovered
this particular formula.
It begins with: 0,1,1,2,3,5,8,13,21,... and runs
onward, with each number the sum of the previous two numbers (8 + 13 =
21, 13 + 21 = 34, etc.). This series is to be found in the reproduction
of male bees, the number of spiral floret formations visible in many
sunflowers, spiraled scales on pine cones and pineapples, the
arrangement of leaves on twigs, as well as many other structures. If you
were to look . downward from above on a tree trunk, you would find that
the branches emerge in accordance with the Fibonacci pattern. One will
issue from the trunk at a certain point, the next one above it will
emerge on a different side of the tree at a point in relation to the
series. Gaze into a sunflower head and you will clearly see the
Fibonacci series in the manner in which the seeds are arranged; there
you see lines spiraling outward. Look sideways at a closed pine cone and
you will see the series spiraling around the cone.
MONARCH AND MILKWEED—The milkweed plant produces
a latex that is sticky and poisonous. Most birds, insects, and animals
avoid it. But the monarch butterfly feeds exclusively on it. Females lay
their eggs on the milkweed, and their larvae feed on the leaves. As they do so, they pack
away the deadly, active ingredient into special sealed-off body cells.
While the poison does the caterpillar no harm, it makes the insect
distasteful to predators. If an inexperienced blue jay eats a monarch,
it immediately vomits it up, and will never again go near that
butterfly.
MONARCH AND VICEROY—The viceroy butterfly looks
strikingly like the monarch, but it lacks two special qualities which
the monarch has: (1) The monarch has the milkweed latex in its body to
protect it against enemies. But the viceroy looks so much like the
monarch that predators leave it alone also, thinking it is a monarch.
(2) The monarch migrates in the fall to the far south, wintering over in
southern California and Mexico. The viceroy dies in the fall.
MAINTAINING BODY HEAT—It is Well known that one
of the special qualities of mammals is that they maintain an even body
temperature. But certain plants do the same. The Philodendron
selloum at certain times
maintains a core temperature of 38 to 46°C (100.4-114.8°F], despite
air temperatures all the way from 4 to 39°C [39.2102.2°F]. Small male
flowers are responsible for equalizing plant temperature. It is thought
that the heat helps the plant diffuse scent and attract insects. Perhaps
there are other reasons.
There is evidence that some insects have organs which
can detect infrared (heat) radiation. At any rate, plant temperature may
be one of the factors attracting them to its flowers.
TREE MECHANICS— Auxins are plant hormones which
determine growth,—where it will occur on the plant and to what extent.
Wherever the auxins flow to, that is where the growth will occur.
In the spring, growth begins in the twigs and
progresses down the stem or trunk. Differences in auxin concentrations
cause trees to grow toward the light, and help the end of a tree that
has been bent over to grow upward.
One scientist, T.A. McMahon, worked out the formula
for the general size and height of trees. The mathematical formula goes
something like this: "The diameter of trees will vary with height
raised to the 3/2 power; that is the length times the square root of the
length." This is a lot of complicated mathematics for a tree to
keep track of, yet somehow it does it. Here is a little more of this
formula: "The mean height trees obtain is only about 25 percent of
that which they could obtain and still not buckle. In other words, in
regard to buckling, trees are designed with a safety factor of about
four."
Another scientist analyzed the knees of cypress
trees, and decided that they provide exactly the type of mechanical
support an engineer would provide for a tree growing in a swamp.
PREPARING FOR WINTER—Plants know that winter is
coming because the weather keeps getting colder. In addition, many, if
not most, also
measure the length of the day. Many flower plants
measure the length of the dark period in every 24hour day. By this they
can know that winter is nearing. Many seeds depend on winter to crack
their seed coats enough to soak up water for sprouting in the spring.
Many tree buds will not open up until after a certain amount of cold
weather. Apple buds need 1,000 to 1,400 hours of near-freezing
temperature before they will open in the spring.
DIATOMS—The humble diatom is
probability one of the
simplest plants in existence. Simple?
It is extremely tiny and mostly made of fragile glass
with many little openings, yet it is almost indestructible. It is
fireproof, yet makes dynamite. It has explosive properties, yet is used
in mines to reduce explosions. It tastes like fish oil, yet is used in
toothpaste. It has no apparent means of locomotion, yet it travels
around by straining its own cytoplasm through one window and out the
other. It looks something like an exquisitely carved pillbox, yet this
pillbox duplicates itself by growing a new lid on the box, and then the
lid grows a new box.
There are over 5,000 different types of diatoms. All
are tiny glass houses; all are intricately marked with design work, yet
no two varieties look exactly alike. It is something like an algae, yet
decidedly different. Each diatom can comfortably live in a thimble-full
of water with 14 million other diatoms.
It moves in the water with the agility of an animal,
yet it is a plant which manufactures chlorophyll and produces oxygen and
food. But it does not produce carbohydrates, as do other plants.
Instead, it produces the oil that give fish a "fishy" smell.
Yet its skeleton is used to refine sugar!
Although one of the smallest of the one-celled
organisms, the diatom recycles 90 percent of the oxygen we breath, and
also provides most of the food for fish and whales. This "simpler
form of life" is so complex in construction that it is used to test
the resolving power of microscope lenses.
ROSE
OF SHARON—This little plant grows in
the dry deserts of Palestine and is not actually a rose but a member of
the mustard family.
Its scientific name, Anastatica, means
"resurrection plant," because when the dried up skeleton of
the plant—nothing more than a dried-up ball of twigs—is immersed in
water, it opens up and extends its branches like a miniature tree.
It begins to bloom in March and April, and by May its
seeds are ripe, but they do not open. They remain dormant, tightly enclosed within little pods
or balls. By that time the leaves have fallen off and the dry, hard,
twigs of the plant have shrunken together and resemble a closed fist.
But that apparently dead plant is all the while
continually measuring rainfall. When some comes, little by little it
releases a few of its seeds. Here is how this complicated action takes
place,—and all done by a plant that appears to be dead:
The seeds are enclosed in a ball. The first part of a
rain causes some of the upper balls to open. If more rain falls right
away, some of the peripheral seeds will drop out. If more rain falls
rather quickly, some more balls will open and drop part of their seeds.
Seeds farther into the center of the cluster of twigs may wait for
decades or even centuries to open.
The twiggy mass is so tightly held together that it
requires rain to expand it. When that happens, then additional rain can
fall on the seed balls and permit them to open and a few seeds to fall.
Additional rain and more seeds will drop out. At any point if the rain
stops, then the twiggy mass will close up again.
It requires 4 millimeters [.157 in] of rain to open
the twig mass, which gradually opens in about 2 hours. When the seeds
fall to the ground, they germinate rapidly—in 8 hours—before the earth
dries out.
This plant is only found in the driest part of
Palestine. In those areas where there is more rainfall, none are found.
This is due to the fact that a small gerbil lives in the wetter areas—and relishes Rose of Sharon seeds.
In nature, everything is in perfect balance.
WALKING "SEEDS" AND "TWIGS"—The
male flowers in oak trees are called catkins.
Sometimes the catkins start walking away! What happens is that a certain
caterpillar feeds on catkins until it is so full of them, that it begins
looking like them! In this way, it avoids being eaten by birds who are
looking for juicy caterpillars, not catkins.
The caterpillars which eat these catkins in the
spring, end up looking like them—even to having fake pollen sacks! But
those caterpillars of that same species, which hatch out in the fall,
also feed in the oak trees—and end up looking like oak twigs! In both
cases it is the same type of caterpiller; the only difference is their
diet.
DIFFICULT LIVING—Some flowers push their way up
through snow and ice, while others lie dormant in the hot sands of the
desert for years, and then spring forth and bloom after a rain that may
come only once in a decade.
Some bacteria can live in hot springs at a
temperature of 175°F [79.4°C], while spores of other bacteria have
survived after being exposed to the temperature of liquid air (-310°F
[-190°C]).
UNUSUAL PLANTS—Bamboo grows all over the world,
yet every so often it dies. No one knows why. When it dies, all the
bamboo plants throughout the world also die, even though separated by
thousands of miles! Then, all over the planet, new sprouts shoot up and
this fast growing plant is seemingly resurrected.
There are several kinds of "air plants"
(epiphytes) that get their nourishment from the oxygen, water, and
minerals they find in the air around them. The staghorn fern is an
example. It grows on other trees, with its leaves pressed against the trunk of the tree to conserve moisture.
Beneath the leaves are large masses of roots which extract nourishment
directly from the atmosphere.
The great water lily of the Amazon and Indonesia has
leaf blades that are five feet in diameter. Some palms have leaves 20
feet [61 dm] long. There are seaweeds that grow 450 feet [137 m] down in
the ocean where there is almost no light.
HELICONIUS AND PASSION
FLOWER— Butterflies of the genus Heliconius only lay their
eggs on the tropical vine, the Passiflora, which is the passion-flower
plant. The vine has features which appear to mimic the distinctive
bright yellow eggs of the butterfly.
Each species of this butterfly lays its eggs on only
one species of passion-flower, so this makes it difficult for the female
butterfly to locate the proper plant. For example, on the island of
Barro Colorado in Panama, there are 1,369 plant species, but only 11 of
them are passion-flower species. So the little butterfly has available
to it only a few of all the plants on the island.
Lawrence E. Gilbert has carefully studied the little
butterflies. Arriving at a passion-flower, the female must figure out if
it is the correct species. Using a specially modified pair of front
legs, it "drums" on the surface of the leaf, trying to figure
out if it is the correct species. Somehow it is able to identify the
plant in this way.
Next, the butterfly must ascertain whether the plant
has room for more eggs. If too many are laid, the plant will later be
stripped of its leaves by the butterfly's offspring—the caterpillars—and
die. The death of that species of passion-flower will bring death to the
type of butterflies depending on it.
So the female must next make "an egg load
assessment." This is a well-documented occurrence not only in
Heliconius butterflies, but other insects as well. As a result of this
survey, the female may lay an egg, or may fly off to check out another
passion-flower plant. Research studies reveal that very few eggs are
ever laid on any one plant. In addition, as part of the
"assessment," the female will check on the possibility that
the plant might be too young. If the eggs are deposited too early, the
hatching caterpillar may devour the shoots before its new leaves appear.
The caterpillars will then only have tough old leaves to eat and will
die from starvation. A lot of careful, yet complicated, thinking must be
done by that tiny insect.
Certain passion-flower species have yellow markings
similar in color to the Heliconius eggs. It was found in greehouse
experiments that eggs were deposited on 5 percent of the plants which
had the yellow markings, compared to 30 percent of those without them.
In another experiment, female butterflies were turned
loose in a greenhouse with plants, some of which already had eggs on
them and some of which didn't. The egg-free plants had new eggs placed
on them 70 percent of the time, whereas only 30 percent of those with
eggs had additional ones deposited. In addition, the butterfly took
twice as long to lay eggs on that 30 percent of the plants, because it
first checked out all the other plants, and finally, in desperation,
laid additional eggs on plants that already had other eggs. But when
this was done, the new eggs were laid on the plant as far as possible
from where other eggs were already on it—to insure that there would be enough food for both clutches of
caterpillars when they hatched.
Pretty smart butterflies; too smart for a creature
that tiny.
Similar studies of butterflies and plants in America
have resulted in similar findings. This would include the swallowtail
butterfly and plants of the genus Aristolochia. So there are a variety
of other insects which go through the difficult decision making process
about plant species, and egg assessment that the Heliconius
must make.
You have just completed
Chapter
12 — The Plants
NEXT—
Go to the next chapter in
this series,
Chapter 13 NATURAL SELECTION
|